Bacterioplankton counting methods
Bacterioplankton counting is the estimation of the abundance of bacterioplankton in a specific body of water, which is useful information to marine microbiologists. Various counting methodologies have been developed over the years to determine the number present in the water being observed. Methods used for counting bacterioplankton include epifluorescence microscopy, flow cytometry, measures of productivity through frequency of dividing cells (FDC), thymine incorporation, and leucine incorporation.
Factors such as salinity, temperature, latitude, various nutrient levels, water movement and the presence of other organisms can affect bacterioplankton enumeration.[1][2][3][4][5] Changes in these factors affect the bacterioplankton count, causing it to vary by body of water, location, distance from shore and season.[6][7][8]
Bacterioplankton count is usually expressed in cells per ml (cells ml−1).
Uses
In understanding marine microbiology and the aquatic ecosystem, bacterioplankton counts can be useful. Observation of bacterioplankton number can provide more information in the following:
Epifluorescence microscopy
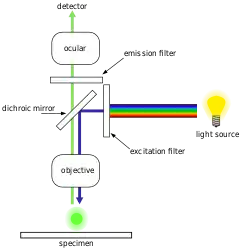
Epifluorescence microscopy is an advanced optical microscope technique that relies on the use of fluorescent dyes that bind to specific biological markers, which then emit a distinctive emission spectra that is identified through the lens. Fluorescent dyes include DAPI, Acridine Orange, SYBR Green 1, and YO-PRO-1, all of which are capable of staining both DNA and RNA structures in biological samples such as bacteria and viruses.[18][19][20][21] However, DNA staining is primarily used for bacterial cell identification. With modern epifluorescence microscopy, the industry standard for estimating and counting bacterial cell quantities is by the use of a DAPI stain.[22] This technique can be performed for samples from a wide range of environments and locations, such as seawater, various sources of freshwater, as well as soils and sediments.[22]
Enumeration technique
In a standard experiment, prepared bacterial samples are placed onto counting slides and then viewed under an epifluorescence microscope. Magnification is set to a level where the 0.1 X 0.1 mm square units on the counting slide are clearly visible.[23] To quantify the bacteria, cells are counted in 5-30 random square unit field-of-views and an average bacteria count per field is tabulated.[22] This value is then extrapolated to estimate the total bacterial cell-count per mL by determining the total number of fields-of-view on the slide deposition area and multiplying this by the average bacterial count per counting unit.[23]
Reliability
To enumerate bacterial cell quantities, only small portions of bacteria in a sample are physically counted for logistical reasons, upon which total abundances are estimated by extrapolation. Mean values are then used for comparison among samples. However the accuracy of this technique, where tabulation of only a small subset is used to estimate total abundance quantities, has been brought into question.[22] Primarily, it has been shown that the distribution of bacterial cells on counting slides can be uneven and inconsistent.[22] In addition, to get a legitimate estimate of bacterial counts by using this technique, it has been suggested that more than 350 individual cells, from 20 fields of view must be measured.[22] This can be not only time consuming, but difficult to achieve in certain samples.
Flow Cytometry

Flow cytometric analysis (or, flow cytometry) is a common procedure in many clinical applications. However, despite its discovery more than three decades ago, its adoption by aquatic microbial ecology in enumeration of bacterioplankton, has been relatively slow.[24] Its use is yet to surpass epifluorescence microscopy.[25] Despite both abundance estimation techniques being relatively accurate, flow cytometry is less prone to human error, more precise, pertains a higher resolution and is capable of examining tens of thousands of cells in a matter of minutes.[24] Flow cytometry is also able to provide information regarding size, activity and morphology of cells besides abundance of cells.[26]
Flow cytometry can be used to distinguish and quantify both photosynthetic and non-photosynthetic bacterioplankton.[26] Quantification of photosynthetic prokaryotes such as cyanobacteria and picoeukaryotic algae is made possible by the ability of photosynthetic pigments to fluoresce.[27] For instance, the different formation of photosynthetic pigments in the two major photosynthetic prokaryotes, prochlorococcus and synechococcus, enable their very distinction.[28][29][30] Prochlorococcus contains divynyl-chlorophylls a and b which display solely red fluorescence under excitation by blue or UV light, while synechococcus emits both orange and red fluorescence; orange from phycobilins and red from chlorophyll. Besides fluorescence, prochlorococcus and synechococus are of significantly different sizes and hence deliver different scatter signals upon flow cytometric analysis. This further helps in their differentiation.[31] Quantification of prochlorococcus is considered a major breakthrough as it has almost only been possible through flow cytometry. This is due to the inability of epifluorescence microscopy to detect the low chlorophyll autofluorescence present in prochlorococcus.[26]
Besides photosynthetic bacterioplankton, non-photosynthetic bacterioplankton can also be enumerated by flow cytometry. This is done via DNA or food vacuole staining.[27] Flow cytometry has especially been successful at differentiating prochloroccocus from heterotrophic bacteria, who's counts were initially confounded due to their similar size.
Use of epifluorescence microscopy over flow cytometry in many microbial ecology labs can be blamed on a number of economic and practical factors. First, the use of commercial flow cytometers requires the expertise of a rigorously trained technician. Second, flow cytometers are fairly expensive in comparison to epi-fluorescence microscopy apparatus. Third, many flow cytometers are designed to examine blood cells; oceanic bacteria are relatively small and hence approach limit of resolution in many commercial flow cytometers.[32]
Enumeration Process
Flow cytometric quantification of bacterioplankton involves four steps: fixation, staining, data processing and data interpretation.
Fixation
Fixation is done to not only preserve sample, but also to increase permeability of cells to stains.[24] However, most common fixation agents have the capacity to alter cells by changing certain aspects such as size, how light is scattered, autofluorescence and nucleic acids. This is problematic as flow cytometric distinction of cells relies on these qualities. Some fixatives also lead to complete loss of cells.[24] Presently, some of the agents used in the fixation process include two variations of formaldehyde (formalin and paraformaldehyde), 70% ethanol, glutaraldehyde and TCA.[33] It is presumed that the best fixation agent for protein and nucleic acids is paraformaldehyde due to its ability to swiftly enter cells.[24]
Staining
In flow cytometry, staining enables distinction of bacterioplankton from non-bacterial particles. It involves the incubation of sample in the wide array of fluorochromes such as UV-excited dyes (DAPI and Hoechst 33342) and blue-light-excited nucleic acid dyes (TO-PRO-1,TOTO-1, SYBR Green I).[31] For a long time, flow cytometers utilized UV-excited dyes to examine bacterioplankton which could be used in either low-cost flow cytometers with limited sensitivity, or expensive flow cytometers with the high sensitivity needed to distinguish heterotrophic bacteria from autotrophs. The introduction of blue-excited dyes such as SYBR Green I, enabled high quality flow cytometric analysis of bacterioplankton on low-cost, high-sensitivity flow cytometers.[31]
Incubation times for optimum staining varies from compound to compound. UV-excited dyes can require an hour or more while blue-light-excited dyes require a mere 15 minutes.[24]
Staining can be accompanied by buffers such as Triton X-100 which make cells more permeable to stains. They are especially used in cell-impermeant dyes like TO-PRO-1. Buffers are also used to dilute dyes sensitive to ionic strength such as Picogreen, YO-PRO-1 and YOYO-1. The use of buffers however, can be harmful to cells as buffers like Triton-X-100 can not only extinguish chlorophyll fluorescence, but also create unwanted background fluorescence. This can increase the difficulty of distinguishing between heterotrophic bacteria and autotrophic prokaryotes.[24]
Counting
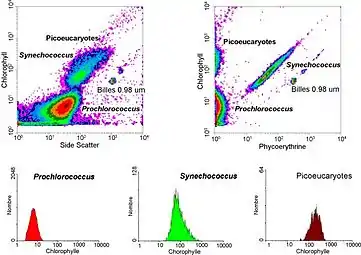
In a flow cytometric analysis, over 200 cells pass in front of a laser beam or mercury lamp every second, a cell at a time. Photomultipliers gather the amount of light each particle scatters and the fluorescence emitted upon excitation. This information is then internalized and interpreted by the system as an event. However, despite the ability of flow cytometers to count cells with very little effort, most have no way of determining actual concentration of cells. This can be determined through a variety of methods including, use of reference beads who's quantity is pre-determined (helps determine the ratio of bacteria to beads), weight measurements before and after experiment and daily calibration of flow.[24]
A big advantage of flow cytometers is their ability to identify different populations of bacterioplanton. This discrimination is done via analysis of four factors; light scatter, green fluorescence, blue fluorescence and red fluorescence. Light scatter analysis is inadequate alone and is often examined alongside fluorescence for a number of reasons; first, sea water contains many particles that scatter light like bacteria. Second, the sizes of many oceanic bacteria approach limit of resolution. The amount of light scattered by cells is determined by not only size of cells, but also internal structure, refractive index, shape and orientation of particle. Scattered light is classified into either forward scatter (FSC) or side scatter (SSC). The former has been associated with cell volume and mass while the latter has been associated with index of refraction, content and granularity of cells [24]
When cell concentrations are higher than 2.5 × 106 cells per ml, the likelihood of more than once cell passing in close proximity and being recorded as a single event is magnified. This is known as coincidence and can be easily avoided by diluting sample before hand[31]
Measures of productivity
Frequency of dividing cells
Frequency of dividing cells (FDC) is a method used to predict the average growth rate of an aquatic heterotrophic bacterial community.[34] The method uses cell division, specifically septum formation, as a proxy for growth rate.[34] Cells are considered divided, when cavities between individual cells (invagination) are observed under epifluorescence microscopy.[34] FDC is based on the assumption that there relationship between the proportion of cells currently dividing and the growth rate in a bacterial community.[35]
Thymidine incorporation
Thymidine incorporation is one of the most extensively used methods to estimate bacterial growth.[36] Thymidine is a precursor for DNA, and DNA synthesis can be measured by tritiated thymidine incorporation into trichloroacetic acid (TCA) – insoluble material via salvage pathways.[37] Thymidine incorporation measures growth based on rates of DNA synthesis, using the assumption that only growing cells can incorporate the radioactive thymidine to synthesize DNA.[38]
Weaknesses of this procedure include labeling of other molecules besides DNA when tritiated thymidine is added to a sample.[36] In cases of carbon limitation, thymidine may also be used as a carbon source instead of as a DNA precursor.[36] Results of thymidine incorporation experiments may be misleading when the proportion of thymidine incorporated into DNA compared to other molecules is not known.[36]
Leucine incorporation
Leucine incorporation is used as a measure of protein synthesis in aquatic bacteria communities.[39] Radio-labeled leucine is added to samples, and its accumulation in hot trichloroacetic acid (CA)-insoluble parts of the cell is determined.[39] The samples are then collected on membrane filter.[39] Leucine protein is taken up by more than 50% of aquatic bacterial populations, and leucine incorporation can be used to estimate nitrogen utilization in the bacterial community.[39]
Marine seasonal succession dynamics
As bacterial populations have unique metabolisms and resource preferences, the use of high-resolution time-series analysis of bacterial compositions allows for the identification of patterns in seasonal bacterial succession.[40] Differences in bacterial community compositions give rise to particular permutations of interspecies bacterial interactions with photosynthetic plankton, protist grazers, and phages thereby impacting seasonality dynamics. Statistical methods used to verify patterns in population dynamics and composition are demonstrated to be replicable over some years, and environmental factors served as predictors of these temporal patterns.[41] Most research activity for bacterioplankton currently occurs in the temperate waters of the northern hemisphere from 30°N to the Arctic Circle at 66°N.[40]
Seasonal succession in temperate regions
As seasonal successions of phytoplankton populations follow a consistent recurring pattern, bacterial dynamics and phytoplankton succession can be correlated.[40] In general, seasonal changes in bacterial composition follow changes in temperature and chlorophyll a, while nutrient availability limits bacterioplankton growth rates.[42][43][44][45][6][46] During water column mixing in late autumn/winter, nutrients brought to the surface kicks start a distinct diatom spring bloom followed by dinoflagellates.[40] After the spring bloom, bacterial production and growth become elevated due to the release of Dissolved organic matter (DOM) from phytoplankton decay.[47][48] In this early succession stage, members of the class Flavobacteria (Bacteroidetes) are typically the dominant components of the bacterial community.[49][50] Genome analysis and meta-transcriptomics have uncovered the presence of bacteria containing multiple hydrolytic enzymes facilitating the degradation and assimilation of DOM.[51][52][53][54] During spring blooms, some members of the Roseobacter clade (Alphaproteobacteria) and some Gammaproteobacteria are usually associated with DOM degradation.[48][49] As temperatures increase and the nutrients from the spring bloom gets depleted, smaller phytoplankton and cyanobacteria grow in the now oligotrophic waters.[40]
As waters become stratified in summer, Roseobacter, SAR86 (Gammaproteobacteria), and SAR11 (Alphaproteobacteria) clades of bacteria increase in abundance.[55][56] The frequently observed autumn diatom and dinoflagellate blooms are correlated with supplementary nutrient inputs and high-frequency sampling in the Baltic Sea found that in autumn, Actinobacteria generally increase followed by different autumn-specific Flavobacteria, SAR11, and Planctomycetes.[49]
In the Mediterranean Sea, deep winter mixing allows members of the SAR11 clade to achieve increased diversity as the oligotrophic populations that once dominated during the summer stratification die off slowly.[57] Among archaea in the Mediterranean Sea, Thaumarchaeota Marine Group I (MGI) and Euryarchaeota Marine Group II (MGII.B) populations became dominant in winter.[58] While in the Baltic Sea, winter mixing brings Epsilon-proteobacteria and archaea populations to the surface from their deep habitat.[49]
References
- Long RA, Azam F (2001-12-05). "Microscale patchiness of bacterioplankton assemblage richness in seawater". Aquatic Microbial Ecology. 26 (2): 103–113. doi:10.3354/ame026103.
- He J, Zhang F, Lin L, Ma Y, Chen J (2012). "Bacterioplankton and picophytoplankton abundance, biomass, and distribution in the Western Canada Basin during summer 2008". Deep Sea Research Part II: Topical Studies in Oceanography. 81-84: 36–45. Bibcode:2012DSRII..81...36H. doi:10.1016/j.dsr2.2012.08.018.
- Wei C, Bao S, Zhu X, Huang X (2008). "Spatio-temporal variations of the bacterioplankton community composition in Chaohu Lake, China". Progress in Natural Science. 18 (9): 1115–1122. doi:10.1016/j.pnsc.2008.04.005.
- López-Flores R, Boix D, Badosa A, Brucet S, Quintana XD (2009). "Environmental factors affecting bacterioplankton and phytoplankton dynamics in confined Mediterranean salt marshes (NE Spain)". Journal of Experimental Marine Biology and Ecology. 369 (2): 118–126. doi:10.1016/j.jembe.2008.11.003.
- Medvinsky, Alexander B.; Adamovich, Boris V.; Aliev, Rubin R.; Chakraborty, Amit; Lukyanova, Elena V.; Mikheyeva, Tamara M.; Nikitina, Ljudmila V.; Nurieva, Nailya I.; Rusakov, Alexey V. (2017). "Temperature as a factor affecting fluctuations and predictability of the abundance of lake bacterioplankton". Ecological Complexity. 32: 90–98. doi:10.1016/j.ecocom.2017.10.002.
- Andersson AF, Riemann L, Bertilsson S (February 2010). "Pyrosequencing reveals contrasting seasonal dynamics of taxa within Baltic Sea bacterioplankton communities". The ISME Journal. 4 (2): 171–81. doi:10.1038/ismej.2009.108. PMID 19829318.
- Ghiglione JF, Murray AE (March 2012). "Pronounced summer to winter differences and higher wintertime richness in coastal Antarctic marine bacterioplankton". Environmental Microbiology. 14 (3): 617–29. doi:10.1111/j.1462-2920.2011.02601.x. PMID 22003839.
- Straza TR, Ducklow HW, Murray AE, Kirchman DL (2010-11-01). "Abundance and single-cell activity of bacterial groups in Antarctic coastal waters". Limnology and Oceanography. 55 (6): 2526–2536. Bibcode:2010LimOc..55.2526S. doi:10.4319/lo.2010.55.6.2526.
- Currie DJ, Kalff J (March 1984). "The relative importance of bacterioplankton and phytoplankton in phosphorus uptake in freshwater1". Limnology and Oceanography. 29 (2): 311–321. Bibcode:1984LimOc..29..311C. doi:10.4319/lo.1984.29.2.0311.
- Lindström ES (December 2001). "Investigating Influential Factors on Bacterioplankton Community Composition: Results from a Field Study of Five Mesotrophic Lakes". Microbial Ecology. 42 (4): 598–605. doi:10.1007/s00248-001-0031-y. PMID 12024242. S2CID 22656746.
- Cotner JB, Biddanda BA (2002-03-01). "Small Players, Large Role: Microbial Influence on Biogeochemical Processes in Pelagic Aquatic Ecosystems". Ecosystems. 5 (2): 105–121. CiteSeerX 10.1.1.484.7337. doi:10.1007/s10021-001-0059-3. S2CID 39074312.
- Harnisz M (March 2013). "Total resistance of native bacteria as an indicator of changes in the water environment". Environmental Pollution. 174: 85–92. doi:10.1016/j.envpol.2012.11.005. PMID 23246751.
- Chen, Xinxin; Wang, Kai; Guo, Annan; Dong, Zhiying; Zhao, Qunfen; Qian, Jie; Zhang, Demin (2016). "Excess phosphate loading shifts bacterioplankton community composition in oligotrophic coastal water microcosms over time". Journal of Experimental Marine Biology and Ecology. 483: 139–146. doi:10.1016/j.jembe.2016.07.009.
- Dai, Wenfang; Zhang, Jinjie; Tu, Qichao; Deng, Ye; Qiu, Qiongfen; Xiong, Jinbo (2017). "Bacterioplankton assembly and interspecies interaction indicating increasing coastal eutrophication". Chemosphere. 177: 317–325. Bibcode:2017Chmsp.177..317D. doi:10.1016/j.chemosphere.2017.03.034. PMID 28319885.
- Urakawa, Hidetoshi; Bernhard, Anne E. (2017). "Wetland management using microbial indicators". Ecological Engineering. 108: 456–476. doi:10.1016/j.ecoleng.2017.07.022.
- Haukka K, Kolmonen E, Hyder R, Hietala J, Vakkilainen K, Kairesalo T, Haario H, Sivonen K (February 2006). "Effect of nutrient loading on bacterioplankton community composition in lake mesocosms". Microbial Ecology. 51 (2): 137–46. doi:10.1007/s00248-005-0049-7. PMID 16435168. S2CID 35399139.
- Zhang D, Wang X, Xiong J, Zhu J, Wang Y, Zhao Q, Chen H, Guo A, Wu J (2014). "Bacterioplankton assemblages as biological indicators of shrimp health status". Ecological Indicators. 38: 218–224. doi:10.1016/j.ecolind.2013.11.002.
- Tanious FA, Veal JM, Buczak H, Ratmeyer LS, Wilson WD (1992-03-31). "DAPI (4',6-diamidino-2-phenylindole) binds differently to DNA and RNA: minor-groove binding at AT sites and intercalation at AU sites". Biochemistry. 31 (12): 3103–3112. doi:10.1021/bi00127a010. PMID 1372825.
- Gonzalez K, Mcvey S, Cunnick J, Udovichenko IP, Takemoto DJ (1995). "Acridine orange differential staining of total DNA and RNA in normal and galactosemic lens epithelial cells in culture using flow cytometry". Current Eye Research. 14 (4): 269–273. doi:10.3109/02713689509033525. PMID 7541739.
- Noble RT, Fuhrman JA (1998-02-13). "Use of SYBR Green I for rapid epifluorescence counts of marine viruses and bacteria". Aquatic Microbial Ecology. 14 (2): 113–118. doi:10.3354/ame014113.
- Marie D, Vaulot D, Partensky F (May 1996). "Application of the novel nucleic acid dyes YOYO-1, YO-PRO-1, and PicoGreen for flow cytometric analysis of marine prokaryotes". Applied and Environmental Microbiology. 62 (5): 1649–55. doi:10.1128/AEM.62.5.1649-1655.1996. PMC 167939. PMID 8633863.
- Muthukrishnan T, Govender A, Dobretsov S, Abed RM (2017-01-08). "Evaluating the Reliability of Counting Bacteria Using Epifluorescence Microscopy". Journal of Marine Science and Engineering. 5 (1): 4. doi:10.3390/jmse5010004.
- O'Connor JT, O'Connor T, Twait R (2009). Water Treatment Plant Performance Evaluations and Operations. John Wiley & Sons, Inc. pp. 193–198. doi:10.1002/9780470431474.app1. ISBN 9780470431474.
- Gasol, Josep M.; Giorgio, Paul A. del (2000-06-30). "Using flow cytometry for counting natural planktonic bacteria and understanding the structure of planktonic bacterial communities". Scientia Marina. 64 (2): 197–224. doi:10.3989/scimar.2000.64n2197. ISSN 1886-8134.
- Giorgio, Paul A. del; Bird, David F.; Prairie, Yves T.; Planas, Dolors (1996-06-01). "Flow cytometric determination of bacterial abundance in lake plankton with the green nucleic acid stain SYTO 13". Limnology and Oceanography. 41 (4): 783–789. Bibcode:1996LimOc..41..783G. doi:10.4319/lo.1996.41.4.0783. ISSN 1939-5590.
- Sieracki, Michael E.; Haugen, Elin M.; Cucci, Terry L. (1995-08-01). "Overestimation of heterotrophic bacteria in the Sargasso Sea: direct evidence by flow and imaging cytometry". Deep Sea Research Part I: Oceanographic Research Papers. 42 (8): 1399–1409. Bibcode:1995DSRI...42.1399S. doi:10.1016/0967-0637(95)00055-B. ISSN 0967-0637.
- Zubkov MV, Burkill PH, Topping JN (2007-01-01). "Flow cytometric enumeration of DNA-stained oceanic planktonic protists". Journal of Plankton Research. 29 (1): 79–86. doi:10.1093/plankt/fbl059.
- WATERBURY, JOHN B.; WATSON, STANLEY W.; GUILLARD, ROBERT R. L.; BRAND, LARRY E. (January 1979). "Widespread occurrence of a unicellular, marine, planktonic, cyanobacterium". Nature. 277 (5694): 293–294. Bibcode:1979Natur.277..293W. doi:10.1038/277293a0. ISSN 1476-4687. S2CID 4270426.
- Chisholm, Sallie W.; Frankel, Sheila L.; Goericke, Ralf; Olson, Robert J.; Palenik, Brian; Waterbury, John B.; West-Johnsrud, Lisa; Zettler, Erik R. (1992-02-01). "Prochlorococcus marinus nov. gen. nov. sp.: an oxyphototrophic marine prokaryote containing divinyl chlorophyll a and b". Archives of Microbiology. 157 (3): 297–300. doi:10.1007/BF00245165. ISSN 0302-8933. S2CID 32682912.
- Chisholm, Sallie W.; Olson, Robert J.; Zettler, Erik R.; Goericke, Ralf; Waterbury, John B.; Welschmeyer, Nicholas A. (July 1988). "A novel free-living prochlorophyte abundant in the oceanic euphotic zone". Nature. 334 (6180): 340–343. Bibcode:1988Natur.334..340C. doi:10.1038/334340a0. ISSN 1476-4687. S2CID 4373102.
- Marie D, Partensky F, Jacquet S, Vaulot D (January 1997). "Enumeration and Cell Cycle Analysis of Natural Populations of Marine Picoplankton by Flow Cytometry Using the Nucleic Acid Stain SYBR Green I". Applied and Environmental Microbiology. 63 (1): 186–93. doi:10.1128/AEM.63.1.186-193.1997. PMC 1389098. PMID 16535483.
- Giorgio PA, Bird DF, Prairie YT, Planas D (June 1996). "Flow cytometric determination of bacterial abundance in lake plankton with the green nucleic acid stain SYTO 13". Limnology and Oceanography. 41 (4): 783–789. Bibcode:1996LimOc..41..783G. doi:10.4319/lo.1996.41.4.0783.
- Rice J, Sleigh MA, Burkill PH, Tarran GA, O'connor CD, Zubkov MV (March 1997). "Flow Cytometric Analysis of Characteristics of Hybridization of Species-Specific Fluorescent Oligonucleotide Probes to rRNA of Marine Nanoflagellates". Applied and Environmental Microbiology. 63 (3): 938–44. doi:10.1128/AEM.63.3.938-944.1997. PMC 1389123. PMID 16535558.
- Hagström A, Larsson U, Hörstedt P, Normark S (May 1979). "Frequency of dividing cells, a new approach to the determination of bacterial growth rates in aquatic environments". Applied and Environmental Microbiology. 37 (5): 805–12. doi:10.1128/AEM.37.5.805-812.1979. PMC 243306. PMID 16345378.
- Newell SY, Christian RR (July 1981). "Frequency of dividing cells as an estimator of bacterial productivity". Applied and Environmental Microbiology. 42 (1): 23–31. doi:10.1128/AEM.42.1.23-31.1981. PMC 243955. PMID 16345812.
- Servais P, Martinez J, Billen G, Vives-Rego J (August 1987). "Determining [H]Thymidine Incorporation into Bacterioplankton DNA: Improvement of the Method by DNase Treatment". Applied and Environmental Microbiology. 53 (8): 1977–9. doi:10.1128/AEM.53.8.1977-1979.1987. PMC 204039. PMID 16347424.
- Bell R, Ahlgren G, Ahlgren I (June 1983). "Estimating Bacterioplankton Production by Measuring [3H]thymidine Incorporation in a Eutrophic Swedish Lake". Applied and Environmental Microbiology. 45 (6): 1709–1721. doi:10.1128/AEM.45.6.1709-1721.1983. PMC 242528. PMID 16346304.
- Fuhrman J, Azam F (July 1980). "Bacterioplankton secondary production estimates for coastal waters of British Columbia, Canada, Antarctica, and California, USA". Applied and Environmental Microbiology. 39 (6): 1085–1095. doi:10.1128/AEM.39.6.1085-1095.1980. PMC 291487. PMID 16345577.
- Kirchman D, K'nees E, Hodson R (March 1985). "Leucine incorporation and its potential as a measure of protein synthesis by bacteria in natural aquatic systems". Applied and Environmental Microbiology. 49 (3): 599–607. doi:10.1128/AEM.49.3.599-607.1985. PMC 373556. PMID 3994368.
- Bunse C, Pinhassi J (June 2017). "Marine Bacterioplankton Seasonal Succession Dynamics". Trends in Microbiology. 25 (6): 494–505. doi:10.1016/j.tim.2016.12.013. PMID 28108182.
- Fuhrman JA, Hewson I, Schwalbach MS, Steele JA, Brown MV, Naeem S (August 2006). "Annually reoccurring bacterial communities are predictable from ocean conditions". Proceedings of the National Academy of Sciences of the United States of America. 103 (35): 13104–9. Bibcode:2006PNAS..10313104F. doi:10.1073/pnas.0602399103. PMC 1559760. PMID 16938845.
- Pinhassi J, Hagström Å (2000-06-15). "Seasonal succession in marine bacterioplankton". Aquatic Microbial Ecology. 21 (3): 245–256. doi:10.3354/ame021245.
- Pinhassi J, Gómez-Consarnau L, Alonso-Sáez L, Sala MM, Vidal M, Pedrós-Alió C, Gasol JM (2006-10-10). "Seasonal changes in bacterioplankton nutrient limitation and their effects on bacterial community composition in the NW Mediterranean Sea". Aquatic Microbial Ecology. 44 (3): 241–252. doi:10.3354/ame044241.
- Sapp M, Wichels A, Wiltshire KH, Gerdts G (March 2007). "Bacterial community dynamics during the winter-spring transition in the North Sea". FEMS Microbiology Ecology. 59 (3): 622–37. doi:10.1111/j.1574-6941.2006.00238.x. PMID 17381518.
- Gilbert JA, Field D, Swift P, Newbold L, Oliver A, Smyth T, Somerfield PJ, Huse S, Joint I (December 2009). "The seasonal structure of microbial communities in the Western English Channel" (PDF). Environmental Microbiology. 11 (12): 3132–9. doi:10.1111/j.1462-2920.2009.02017.x. hdl:1912/3133. PMID 19659500.
- Gilbert JA, Steele JA, Caporaso JG, Steinbrück L, Reeder J, Temperton B, Huse S, McHardy AC, Knight R, Joint I, Somerfield P, Fuhrman JA, Field D (February 2012). "Defining seasonal marine microbial community dynamics". The ISME Journal. 6 (2): 298–308. doi:10.1038/ismej.2011.107. PMC 3260500. PMID 21850055.
- Riemann L, Steward GF, Azam F (February 2000). "Dynamics of bacterial community composition and activity during a mesocosm diatom bloom". Applied and Environmental Microbiology. 66 (2): 578–87. doi:10.1128/AEM.66.2.578-587.2000. PMC 91866. PMID 10653721.
- Buchan A, LeCleir GR, Gulvik CA, González JM (October 2014). "Master recyclers: features and functions of bacteria associated with phytoplankton blooms". Nature Reviews. Microbiology. 12 (10): 686–98. doi:10.1038/nrmicro3326. PMID 25134618. S2CID 26684717.
- Lindh MV, Sjöstedt J, Andersson AF, Baltar F, Hugerth LW, Lundin D, Muthusamy S, Legrand C, Pinhassi J (July 2015). "Disentangling seasonal bacterioplankton population dynamics by high-frequency sampling". Environmental Microbiology. 17 (7): 2459–76. doi:10.1111/1462-2920.12720. PMID 25403576.
- Alderkamp A, Sintes E, Herndl GJ (2006-12-21). "Abundance and activity of major groups of prokaryotic plankton in the coastal North Sea during spring and summer". Aquatic Microbial Ecology. 45 (3): 237–246. doi:10.3354/ame045237.
- Fernández-Gómez B, Richter M, Schüler M, Pinhassi J, Acinas SG, González JM, Pedrós-Alió C (May 2013). "Ecology of marine Bacteroidetes: a comparative genomics approach". The ISME Journal. 7 (5): 1026–37. doi:10.1038/ismej.2012.169. PMC 3635232. PMID 23303374.
- Teeling H, Fuchs BM, Becher D, Klockow C, Gardebrecht A, Bennke CM, Kassabgy M, Huang S, Mann AJ, Waldmann J, Weber M, Klindworth A, Otto A, Lange J, Bernhardt J, Reinsch C, Hecker M, Peplies J, Bockelmann FD, Callies U, Gerdts G, Wichels A, Wiltshire KH, Glöckner FO, Schweder T, Amann R (May 2012). "Substrate-controlled succession of marine bacterioplankton populations induced by a phytoplankton bloom". Science. 336 (6081): 608–11. Bibcode:2012Sci...336..608T. doi:10.1126/science.1218344. PMID 22556258. S2CID 29249533.
- Teeling H, Fuchs BM, Bennke CM, Krüger K, Chafee M, Kappelmann L, Reintjes G, Waldmann J, Quast C, Glöckner FO, Lucas J, Wichels A, Gerdts G, Wiltshire KH, Amann RI (April 2016). "Recurring patterns in bacterioplankton dynamics during coastal spring algae blooms". eLife. 5: e11888. doi:10.7554/eLife.11888. PMC 4829426. PMID 27054497.
- Taylor JD, Cottingham SD, Billinge J, Cunliffe M (January 2014). "Seasonal microbial community dynamics correlate with phytoplankton-derived polysaccharides in surface coastal waters". The ISME Journal. 8 (1): 245–8. doi:10.1038/ismej.2013.178. PMC 3869024. PMID 24132076.
- Agawin NS, Duarte CM, Agustí S (1998-09-03). "Growth and abundance of Synechococcus sp. in a Mediterranean Bay: seasonality and relationship with temperature". Marine Ecology Progress Series. 170: 45–53. Bibcode:1998MEPS..170...45A. doi:10.3354/meps170045.
- Alonso-Sáez L, Balagué V, Sà EL, Sánchez O, González JM, Pinhassi J, Massana R, Pernthaler J, Pedrós-Alió C, Gasol JM (April 2007). "Seasonality in bacterial diversity in north-west Mediterranean coastal waters: assessment through clone libraries, fingerprinting and FISH". FEMS Microbiology Ecology. 60 (1): 98–112. doi:10.1111/j.1574-6941.2006.00276.x. PMID 17250750.
- Salter I, Galand PE, Fagervold SK, Lebaron P, Obernosterer I, Oliver MJ, Suzuki MT, Tricoire C (February 2015). "Seasonal dynamics of active SAR11 ecotypes in the oligotrophic Northwest Mediterranean Sea". The ISME Journal. 9 (2): 347–60. doi:10.1038/ismej.2014.129. PMC 4303628. PMID 25238399.
- Hugoni M, Taib N, Debroas D, Domaizon I, Jouan Dufournel I, Bronner G, Salter I, Agogué H, Mary I, Galand PE (April 2013). "Structure of the rare archaeal biosphere and seasonal dynamics of active ecotypes in surface coastal waters". Proceedings of the National Academy of Sciences of the United States of America. 110 (15): 6004–9. Bibcode:2013PNAS..110.6004H. doi:10.1073/pnas.1216863110. PMC 3625260. PMID 23536290.