Chiral Lewis acid
Chiral Lewis acids (CLAs) are a type of Lewis acid catalyst that effects the chirality of the substrate as it reacts with it. In such reactions, the synthesis favors the formation of a specific enantiomer or diastereomer. The method then is an enantioselective asymmetric synthesis reaction. Since they affect chirality, they produce optically active products from optically inactive or mixed starting materials. This type of preferential formation of one enantiomer or diastereomer over the other is formally known as an asymmetric induction. In this kind of Lewis acid. the electron-accepting atom is typically a metal, such as indium, zinc, lithium, aluminium, titanium, or boron. The chiral-altering ligands employed for synthesizing these acids most often have multiple Lewis basic sites (often a diol or a dinitrogen structure) that allow the formation of a ring structure involving the metal atom.[1][2]
Achiral Lewis acids have been used for decades to promote the synthesis of racemic mixtures in a myriad different reactions. Since the 1960s, chemists have used chiral Lewis acids to induce enantioselective reactions. This is useful when the desired product is a specific enantiomer, common in drug synthesis. Common reaction types include Diels-Alder reactions, the ene reaction, [2+2] cycloaddition reactions, hydrocyanation of aldehydes, and most notably, Sharpless epoxidations.[3]
Theory
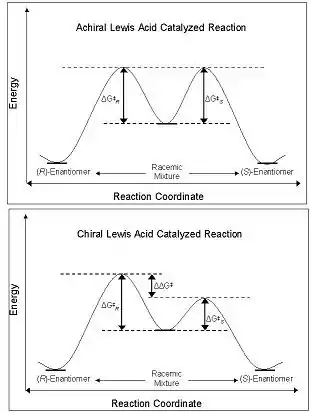
The enantioselectivity of CLAs derives from their ability to perturb the free energy barrier along the reaction coordinate pathway that leads to either the R- or S- enantiomer. Ground state diastereomers and enantiomers are of equal energy in the ground state, and when reacted with an achiral lewis acid, their diastereomeric intermediates, transition states, and products are also of equal energy. This leads to the production of racemic mixtures of products. However, when a CLA is used in the same reaction, the energetic barrier of formation of one diastereomer is less than that of another – the reaction is under kinetic control. If the difference in the energy barriers between the diastereomeric transition states are of sufficient magnitude, then a high enantiomeric excess of one isomer should be observed (Figure 2).[4]
Applications of CLAs in asymmetric synthesis
Diels-Alder reaction
Diels-Alder reactions occur between a conjugated diene and an alkene (commonly known as the dienophile). This cycloaddition process allows for the stereoselective formation of cyclohexene rings capable of possessing as many as four contiguous stereogenic centers.
Diels-Alder reactions can lead to formation of a variety of structural isomers and stereoisomers. The molecular orbital theory considers that endo transition state, instead of the exo transition state, is favored (endo addition rule). Also, augmented secondary orbital interactions have been postulated as the source of enhanced endo diastereoselection.
The addition of a CLA selectively activates one component of the reaction (the diene or dienophile) while providing a stereodefined environment that permits unique enantioselectivity.
Koga and coworkers disclosed the first practical example of a catalytic enantioselective Diels-Alder reaction promoted by a CLA - menthoxyaluminum dichloride - derived from menthol and ethylaluminum dichloride.[5]
A decade later, Elias James Corey introduced an effective aluminum-diamine controller for Diels-Alder reaction. Formation of the active catalyst is achieved by treatment of the bis(sulfonamide) with trimethylaluminum; recovery of the ligand was essentially quantitative. The proposed tetracoordinate aluminum prevent the imide acting as a chelating Lewis base, while enhance the α-vinyl proton of the dienophile and the benzylic proton of the catalyst.
The X-ray structure of the catalyst showed a stereodefined environment.[6]
In 1993, Wulff and coworkers found a complex derived from diethylaluminium chloride and a “vaulted” biaryl ligand below catalyzed the enantioselective Diels-Alder reaction between cyclopentadiene and methacrolein. The chiral ligand is recovered quantitatively by silica gel chromatography.[7]
Hisashi Yamamoto and coworkers have developed a practical Diels-Alder catalyst for aldehyde dienophiles. The chiral (acyloxy) borane (CAB) complex is effective in catalyzing a number of aldehyde Diels-Alder reactions. NMR spectroscopic experiments indicated close proximity of the aldehyde and the aryl ring. Also, Pi stacking between the aryl group and aldehyde was suggested as an organizational feature which imparted high enantioselectivity to the cycloaddition.[8]
Yamamoto and co-workers have introduced a conceptually interesting series of catalysts that incorporate an acidic proton into the active catalyst. This kind of what so called Bronsted acid-assisted chiral Lewis acid (BLA) catalyzes a number of diene-aldehyde cycloaddition reactions.[9]
Aldol reaction
In the aldol reaction, the diastereoselectivity of the product is often dictated by the geometry of the enolate according to the Zimmerman-Traxler model. The model predicts that the Z enolate will give syn products and that E enolates will give anti products. Chiral Lewis acids allow products that defy the Zimmerman-Traxler model, and allows for control of absolute stereochemistry. Kobayashi and Horibe demonstrated this in the synthesis of dihydroxy thioester derivatives, using a tin-based chiral Lewis acid.[10]
The transition structures for reactions with both the R and S catalyst enantiomers are shown below.
Baylis-Hillman Reaction
The Baylis-Hillman reaction is a route for C-C bond formation between an alpha, beta-unsaturated carbonyl and an aldehyde, which requires a nucleophilic catalyst, usually a tertiary amine, for a Michael-type addition and elimination. The stereoselectivity of these reactions is usually poor. Chen et al. demonstrated an enantioselective chiral Lewis acid-catalyzed reaction. Lanthanum was used in this case. Similarly a chiral amine may also be used to achieve stereoselectivity.[11]
The product obtained by the reaction using the chiral catalyst was obtained in good yield with excellent enantioselectivity.
Ene reaction
Chiral lewis acids have also proven useful in the ene reaction. When catalyzed by an achiral lewis acid the reaction normally provides good diastereoselectivity.[12]
When a chiral lewis acid catalyst was used good enantioselectivity was observed.
The enantioselectivity is believed to be due to the steric interactions between the methyl and phenyl group, which makes the transition structure of the iso product considerably more favorable.
Utility of chiral Lewis acids
Asymmetric synthesis and production of enantiomerically pure substances through the use of CLAs is of particular interest to organic chemists and pharmaceutical corporations. Because many pharmaceuticals target enzymes which are specific for a particular enantiomer, compounds intended for patient administration must be of a high optical purity. Furthermore, resolution of a particular enantiomer from a racemic mixture is both costly and wasteful.
Notes
- Lewis Acid Reagents. A Practical Approach. Yamamoto, H., Oxford University Press. 1999 (accessed December 3, 2008)
- Bin, Y., Pikul, S., Imwinkelried, R., Corey, E.J. 1989, JACS, (14) 5493-5495
- Narasaka, K. Synthesis. 1991 (01) 1-11
- Morrison, J.D., Mosher, H.S. (1971). Asymmetric Organic Reactions. Prentice-Hall, Inc. ISBN 978-0-13-049551-8.CS1 maint: multiple names: authors list (link)
- Hashimoto S-I, Komeshima N, Koga K, 1979, J Chem Soc Chem Commun, 437
- Coery, EJ; Sarshar, S; Bordner, J, 1992, J Am Chem Soc, 114, 7938
- Bao, J; Wulff, WD; Rheingold, AL, 1993, J Am Chem Soc, 115, 3814
- Ishihara, K; Gao, Q; Yamamoto, H, 1993, J Am Chem Soc, 115, 10412
- Ishihara, K; Yamamoto, H, 1994, J Am Chem Soc, 116, 1561
-
- Kobayashi, S.; Horibe, M., 1997, Chem. Eur. J., 3, 9, 1472-1481
- Yang, K.; Lee, W.; Pan, J.; Chen, K., 2003, J. Org. Chem., 68, 915-919
- Yang, D.; Yang, M.; Zhu, N., 2003 Org. Lett., 5, 20, 3749-3752
-
- Kimura, M.; Ezoe, A,; Mori, M.; Iwata, K.; Tamaru., Y., 2006, JACS, 128, 8559-8568
References
- Lewis Acid Reagents. A Practical Approach. Yamamoto, H., Oxford University Press., 1999
- Bin, Y., Pikul, S., Imwinkelried, R., Corey, E.J. 1989, JACS, (14) 5493-5495
- Narasaka, K. 1991, Synthesis, (01) 1-11
- Asymmetric Organic Reactions. Morrison, J.D., Mosher, H.S. Prentice-Hall, Inc., 1971