Host microbe interactions in Caenorhabditis elegans
Caenorhabditis elegans- microbe interactions are defined as any interaction that encompasses the association with microbes that temporarily or permanently live in or on the nematode C. elegans. The microbes can engage in a commensal, mutualistic or pathogenic interaction with the host. These include bacterial, viral, unicellular eukaryotic, and fungal interactions. In nature C. elegans harbours a variety of different microbes. In contrast, C. elegans strains that are cultivated in laboratories for research purposes have lost the natural associated microbial communities and are commonly maintained on a single bacterial strain, Escherichia coli OP50. However, E. coli OP50 does not allow for reverse genetic screens because RNAi libraries have only been generated in strain HT115. This limits the ability to study bacterial effects on host phenotypes.[1] The host microbe interactions of C. elegans are closely studied because of their orthologs in humans.[1] Therefore, the better we understand the host interactions of C. elegans the better we can understand the host interactions within the human body.

Natural ecology

C. elegans is a well-established model organism in different research fields, yet its ecology however is only poorly understood. They have a short development cycle only lasting three days with a total life span of about two weeks.[1]C. elegans were previously considered a soil-living nematode,[2][3][4] but in the last 10 years it was shown that natural habitats of C. elegans are microbe-rich, such as compost heaps, rotten plant material, and rotten fruits.[2][5][6][7][8] Most of the studies on C. elegans are based on the N2 strain, which has adapted to laboratory conditions.[9][10][11] Only in the last few years the natural ecology of C. elegans has been studied in more detail[12] and one current research focus is its interaction with microbes.[13] As C. elegans feeds on bacteria (microbivory), the intestine of worms isolated from the wild is usually filled with a large number of bacteria.[8][14][15] In contrast to the very high diversity of bacteria in the natural habitat of C. elegans, the lab strains are only fed with one bacterial strain, the Escherichia coli derivate OP50 .[16] OP50 was not co-isolated with C. elegans from nature, but was rather used because of its high convenience for laboratory maintenance.[17] Bleaching is a common method in the laboratory to clean C. elegans of contaminations and to synchronize a population of worms.[18] During bleaching the worms are treated with 5N NaOH and household bleach, leading to the death of all worms and survival of only the nematode eggs.[18] The larvae hatching from these eggs lack any microbes, as none of the currently known C. elegans-associated microbes can be transferred vertically. Since most laboratory strains are kept under these gnotobiotic conditions, nothing is known about the composition of the C. elegans microbiota.[19] The ecology of C. elegans can only be fully understood in the light of the multiple interactions with the microorganisms, which it encounters in the wild. The effect of microbes on C. elegans can vary from beneficial to lethal.
Beneficial microbes
In its natural habitat C. elegans is constantly confronted with a variety of bacteria that could have both negative and positive effects on its fitness. To date, most research on C. elegans-microbe interactions focused on interactions with pathogens. Only recently, some studies addressed the role of commensal and mutualistic bacteria on C. elegans fitness. In these studies, C. elegans was exposed to various soil bacteria, either isolated in a different context or from C. elegans lab strains transferred to soil.[20][21] These bacteria can affect C. elegans either directly through specific metabolites, or they can cause a change in the environmental conditions and thus induce a physiological response in the host.[20] Beneficial bacteria can have a positive effect on the lifespan, generate certain pathogen resistances, or influence the development of C. elegans.
Lifespan extension

The lifespan of C. elegans is prolonged when grown on plates with Pseudomonas sp. or Bacillus megaterium compared to individuals living on E.coli.[20] The lifespan extension mediated by B. megaterium is greater than that caused by Pseudomonas sp.. As determined by microarray analysis (a method, which allows the identification of C. elegans genes that are differentially expressed in response to different bacteria), 14 immune defence genes were up-regulated when C. elegans was grown on B. megaterium, while only two were up-regulated when fed with Pseudomonas sp. In addition to immune defence genes, other upregulated genes are involved in the synthesis of collagen and other cuticle components, indicating that the cuticle might play an important role in the interaction with microbes. Although some of the genes are known to be important for C. elegans lifespan extension, the precise underlying mechanisms still remain unclear.[20]
Protection against microbes
The microbial communities residing inside the host body have now been recognized to be important for effective immune responses.[21] Yet the molecular mechanisms underlying this protection are largely unknown. Bacteria can help the host to fight against pathogens either by directly stimulating the immune response or by competing with the pathogenic bacteria for available resources.[22][23] In C. elegans, some associated bacteria seem to generate protection against pathogens. For example, when C. elegans is grown on Bacillus megaterium or Pseudomonas mendocina, worms are more resistant to infection with the pathogenic bacterium Pseudomonas aeruginosa [21], which is a common bacterium in C. elegans’ natural environment and therefore a potential natural pathogen.[24] This protection is characterized by prolonged survival on P. aeruginosa in combination with a delayed colonization of C. elegans by the pathogen. Due to its comparatively large size B. megaterium is not an optimal food source for C. elegans,[25] resulting in a delayed development and a reduced reproductive rate. The ability of B. megaterium to enhance resistance against the infection with P. aeruginosa seems to be linked to the decrease in reproductive rate. However, the protection against P. aeruginosa infection provided by P. mendocina is reproduction independent, and depends on the p38 mitogen-activated protein kinase pathway. P. mendocina is able to activate the p38 MAPK pathway and thus to stimulate the immune response of C. elegans against the pathogen.[21] A common way for an organism to protect itself against microbes is to increase fecundation to increase the surviving individuals in the face of an attack. This defense against parasites are genetically linked to stress response pathways and dependent on the innate immune system.[26]
Effects on development
Under natural conditions it might be advantageous for C. elegans to develop as fast as possible to be able to reproduce rapidly. The bacterium Comamonas DA1877 accelerates the development of C. elegans.[27] Neither TOR (target of rapamycin), nor insulin signalling seem to mediate this effect on the accelerated development. It is thus possible that secreted metabolites of Comamonas, which might be sensed by C. elegans, lead to faster development. Worms that were fed with Comamonas DA1877 also showed a reduced number of offspring and a reduced lifespan.[27][28] Another microbe that accelerates C. elegans' growth are L . sphaericus. This bacteria significantly increased the growth rate of C. elegans when compared to their normal diet of E. coli OP50.[29] C. elegans are mostly grown and observed in a controlled laboratory with a controlled diet, therefore, they may show differential growth rates with naturally occurring microbes.
Pathogenic microbes
In its natural environment C. elegans is confronted with a variety of different potential pathogens. C. elegans has been used intensively as a model organism for studying host-pathogen interactions and the immune system.[4][30] These studies revealed that C. elegans has well-functioning innate immune defenses. The first line of defense is the extremely tough cuticle that provides an external barrier against pathogen invasion.[31] In addition, several conserved signaling pathways contribute to defense, including the DAF-2/DAF-16 insulin-like receptor pathway and several MAP kinase pathways, which activate physiological immune responses.[32] Finally, pathogen avoidance behavior represents another line of C. elegans immune defense.[33] All these defense mechanisms do not work independently, but jointly to ensure an optimal defense response against pathogens.[30] Many microorganisms were found to be pathogenic for C. elegans under laboratory conditions. To identify potential C. elegans pathogens, worms in the L4 larval stage are transferred to a medium that contains the organism of interest, which is a bacterium in most cases. Pathogenicity of the organism can be inferred by measuring the lifespan of worms. There are several known human pathogens that have a negative effect on C. elegans survival. However, only very few natural C. elegans pathogens are currently known.[4]
Eukaryotic microbes
One of the best studied natural pathogens of C. elegans is the microsporidium Nematocida parisii, which was directly isolated from wild-caught C. elegans. N. parisii is an intracellular parasite that is exclusively transmitted horizontally from one animal to another. The microsporidian spores are likely to exit the cells by disrupting a conserved cytoskeletal structure in the intestine called the terminal web. It seems that none of the known immune pathways of C. elegans is involved in mediating resistance against N. parisii. Microsporidia were found in several nematodes isolated from different locations, indicating that microsporidia are common natural parasites of C. elegans. The N. parisii-C. elegans system represents a very useful tool to study infection mechanisms of intracellular parasites.[4] Additionally, a new species of microsporidia was recently found in a wild caught C. elegans that genome sequencing places in the same genus Nematocida as prior microsporidia seen in these nematodes. This new species was named Nematocida displodere, after a phenotype seen in late infected worms that explode at the vulva to release infectious spores. N. displodere was shown to infect a broad range of tissues and cell types in C. elegans, including the epidermis, muscle, neurons, intestine, seam cells, and coelomocytes. Strangely, the majority of intestinal infection fails to grow to later parasite stages, while the muscle and epidermal infection thrives.[34] This is in stark contrast to N. parisii which infects and completes its entire life cycle in the C. elegans intestine. These related Nematocida species are being used to study the host and pathogen mechanisms responsible for allowing or blocking eukaryotic parasite growth in different tissue niches. Another eukaryotic pathogen is the fungus Drechmeria coniospora, which has not been directly co-isolated with C. elegans from nature, but is still considered to be a natural pathogen of C. elegans. D. coniospora attaches to the cuticle of the worm at the vulva, mouth, and anus and its hyphae penetrate the cuticle. In this way D. coniospora infects the worm from the outside, while the majority of bacterial pathogens infect the worm from the intestinal lumen.[35][36]
Viral pathogens
In 2011 the first naturally associated virus was isolated from C. elegans found outside of a laboratory. The Orsay virus is an RNA virus that is closely related to nodaviruses. The virus is not stably integrated into the host genome. It is transmitted horizontally under laboratory conditions. An antiviral RNAi pathway is essential for C. elegans resistance against Orsay virus infection.[37] To date there has not been a virus, other intracellular pathogens, or multicellular parasite that have been able to affect the nematode. Because of this we cannot use C. elegans as an experimental system for these interactions. In 2005, two reports have shown that vesicular stomatitis virus (VSV), an arbovirus with a many invertebrate and vertebrate host range, could replicate in primary cells derived from C. elegans embryos.[38]
Bacterial pathogens
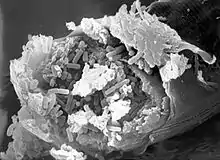
Two bacterial strains of the genus Leucobacter were co-isolated from nature with the two Caenorhabditis species C. briggsae and C. n. spp 11, and named Verde 1 and Verde 2. These two Leucobacter strains showed contrasting pathogenic effects in C. elegans. Worms that were infected with Verde 2 produced a deformed anal region (“Dar” phenotype), while infections with Verde 1 resulted in slower growth due to coating of the cuticle with the bacterial strain. In liquid culture Verde 1 infected worms stuck together with their tails and formed so called “worm stars”. The trapped worms cannot free themselves and eventually die. After death C. elegans is then used as a food source for the bacteria. Only larvae in the L4 stage seem to be able to escape by autotomy. They split their bodies into half, so that the anterior half can escape. The “half-worms” remain viable for several days.[39] The Gram-positive bacterium Bacillus thuringiensis is likely associated with C. elegans in nature. B. thuringiensis is a soil bacterium that is often used in infection experiments with C. elegans.[40][41] It produces spore-forming toxins, called crystal (Cry) toxins, which are associated with spores. These are jointly taken up by C. elegans orally. Inside the host, the toxins bind to the surface of intestinal cells, where the formation of pores in intestinal cells is induced, causing their destruction. The resulting change in milieu in the gut leads to germination of the spores, which subsequently proliferate in the worm body.[42][43][44] An aspect of the C. elegans–B. thuringiensis system is the high variability in pathogenicity between different strains.[41][44] There are highly pathogenic strains, but also strains that are less or even non-pathogenic.[41][44]
References
- Zhang, Jingyan; Holdorf, Amy D.; Walhout, Albertha J.M. (August 2017). "C. elegans and its bacterial diet as a model for systems-level understanding of host-microbiota interactions". Current Opinion in Biotechnology. 46: 74–80. doi:10.1016/j.copbio.2017.01.008. ISSN 0958-1669. PMC 5544573. PMID 28189107.
- Haber, M; Schüngel, M; Putz, A; Müller, S; Hasert, B; Schulenburg, H (2004). "Evolutionary history of Caenorhabditis elegans inferred from microsatellites: evidence for spatial and temporal genetic differentiation and the occurrence of outbreeding". Mol Biol Evol. 22 (1): 160–173. doi:10.1093/molbev/msh264. PMID 15371529.
- Nørhave, NJ; Spurgeon, D; Svendsen, C; Cedergreen, N (2012). "How does growth temperature affect cadmium toxicity measured on different life history traits in the soil nematode Caenorhabditis elegans?". Environ Toxicol Chem. 31 (4): 787–793. doi:10.1002/etc.1746. PMID 22253140.
- Troemel, ER; Félix, M-A; Whiteman, NK; Barrière, A; Ausubel, FM (2008). "Microsporidia are natural intracellular parasites of the nematode Caenorhabditis elegans". PLOS Biol. 6 (12): 2736–2752. doi:10.1371/journal.pbio.0060309. PMC 2596862. PMID 19071962.
- Barrière A, Félix MA (2007). "Temporal dynamics and linkage disequilibrium in natural Caenorhabditis elegans populations". Genetics. 176 (2): 999–1011. doi:10.1534/genetics.106.067223. PMC 1894625. PMID 17409084.
- Kiontke KC, Félix MA, Ailion M, Rockman MV, Braendle C, Pénigault JB, Fitch DH (2011). "A phylogeny and molecular barcodes for Caenorhabditis, with numerous new species from rotting fruits". BMC Evol Biol. 11: 339. doi:10.1186/1471-2148-11-339. PMC 3277298. PMID 22103856.
- Blaxter M, Denver DR (2012). "The worm in the world and the world in the worm". BMC Biol. 10: 57. doi:10.1186/1741-7007-10-57. PMC 3382423. PMID 22731915.
- Félix MA, Duveau F (2012). "Population dynamics and habitat sharing of natural populations of Caenorhabditis elegans and C. briggsae". BMC Biol. 10: 59. doi:10.1186/1741-7007-10-59. PMC 3414772. PMID 22731941.
- McGrath PT, Xu Y, Ailion M, Garrison JL, Butcher RA, Bargmann CI (2011). "Parallel evolution of domesticated Caenorhabditis species targets pheromone receptor genes". Nature. 477 (7364): 321–325. Bibcode:2011Natur.477..321M. doi:10.1038/nature10378. PMC 3257054. PMID 21849976.
- Weber KP, De S, Kozarewa I, Turner DJ, Babu MM, de Bono M (2010). "Whole Genome Sequencing Highlights Genetic Changes Associated with Laboratory Domestication of C. elegans". PLOS ONE. 5 (11): e13922. Bibcode:2010PLoSO...513922W. doi:10.1371/journal.pone.0013922. PMC 2978686. PMID 21085631.
- Barrière, A.; Félix, M.-A. (2005). "Natural variation and population genetics of Caenorhabditis elegans (December 26, 2005), WormBook, ed. The C. elegans Research Community". WormBook: 1–19. doi:10.1895/wormbook.1.43.1. PMC 4781346. PMID 18050391.
- Félix, M.-A.; Barrière, A. (2010). "The natural history of Caenorhabditis elegans". Current Biology. 20 (22): R965–9. doi:10.1016/j.cub.2010.09.050. PMID 21093785. S2CID 12869939.
- Silvia DM, Furhmann JJ, Hartel PG, Zuberer DA. "Principles and Applications of Soil Microbiology (1998) (Prentice Hall, Upper Saddle River, NJ)". Cite journal requires
|journal=
(help) - Garigan D, Hsu AL, Fraser AG, Kamath RS, Ahringer J, Kenyon C (2002). "Genetic analysis of tissue aging in Caenorhabditis elegans: a role for heat-shock factor and bacterial proliferation". Genetics. 161 (3): 1101–1112. PMC 1462187. PMID 12136014.
- McGee MD, Weber D, Day N, Vitelli C, Crippen D, Herndon LA, Hall DH, Melov S (2011). "Loss of intestinal nuclei and intestinal integrity in aging C. elegans". Aging Cell. 10 (4): 699–710. doi:10.1111/j.1474-9726.2011.00713.x. PMC 3135675. PMID 21501374.
- Brenner, S. (1974). "The genetics of Caenorhabditis elegans". Genetics. 77 (1): 71–94. PMC 1213120. PMID 4366476.
- Frézal, L., Félix, M.A. "The natural History of model organisms -C. elegans outside the Petri dish. (2015) elifescience.org". Cite journal requires
|journal=
(help)CS1 maint: multiple names: authors list (link) - Stiernagle, T. (February 11, 2006). The C. elegans Research Community (ed.). "Maintenance of C. elegans". WormBook: 1–11. doi:10.1895/wormbook.1.101.1. PMC 4781397. PMID 18050451.
- Clark, L.C., Hodgkin, J. (2014). "Commensals, probiotics and pathogens in the C aenorhabditis elegans model". Cell Microbiol. 16 (1): 27–38. doi:10.1111/cmi.12234. PMID 24168639. S2CID 3520862.CS1 maint: multiple names: authors list (link)
- Coolon, J.D., Jones, K.L., Todd, T.C., Carr, B.C., and Herman, M.A. (2009). "Caenorhabditis elegans Genomic Response to Soil Bacteria Predicts Environment-Specific Genetic Effects on Life History Traits". PLOS Genetics. 5 (6): e1000503. doi:10.1371/journal.pgen.1000503. PMC 2684633. PMID 19503598.CS1 maint: multiple names: authors list (link)
- Montalvo-Katz, Sirena; Huang, Hao; Appel, Michael David; Berg, Maureen; Shapira, Michael (2012). "Association with Soil Bacteria Enhances p38-Dependent Infection Resistance in Caenorhabditis elegans". Infection and Immunity. 81 (2): 514–520. doi:10.1128/IAI.00653-12. PMC 3553824. PMID 23230286.
- Sekirov I, Russell SL, Antunes LC, Finlay BB (2010). "Gut microbiota in health and disease. Physiol. Rev". Physiological Reviews. 90 (3): 859–904. doi:10.1152/physrev.00045.2009. PMID 20664075. S2CID 9281721.
- Stecher B, Hardt WD (2011). "Mechanisms controlling pathogen colonization of the gut". Curr. Opin. Microbiol. 14 (1): 82–91. doi:10.1016/j.mib.2010.10.003. PMID 21036098.
- Tan MW, Mahajan-Miklos S, Ausubel FM (1999). "Killing of Caenorhabditis elegans by Pseudomonas aeruginosa used to model mammalian bacterial pathogenesis". Proc. Natl. Acad. Sci. U.S.A. 96 (2): 715–720. Bibcode:1999PNAS...96..715T. doi:10.1073/pnas.96.2.715. PMC 15202. PMID 9892699.
- Avery L, Shtonda BB (2003). "Food transport in the C. elegans pharynx". J. Exp. Biol. 206 (14): 2441–2457. doi:10.1242/jeb.00433. PMC 3951750. PMID 12796460.
- Pike, Victoria L.; Ford, Suzanne A.; King, Kayla C.; Rafaluk‐Mohr, Charlotte (2019-10-01). "Fecundity compensation is dependent on the generalized stress response in a nematode host". Ecology and Evolution. 9 (20): 11957–11961. doi:10.1002/ece3.5704. ISSN 2045-7758. PMC 6822023. PMID 31695900.
- MacNeil, L.T., Watson, E., Arda, H.E., Zhu, L.J., and Walhout, A.J.M (2013). "Diet-induced developmental acceleration independent of TOR and insulin in C. elegans". Cell. 153 (1): 240–252. doi:10.1016/j.cell.2013.02.049. PMC 3821073. PMID 23540701.CS1 maint: multiple names: authors list (link)
- Altun, Z.F.; Hall, D.H. "Introduction. In WormAtlas". Cite journal requires
|journal=
(help) - Go, Junhyeok (2014). "Extended longevity and robust early-stage development of Caenorhabditis elegans by a soil microbe, Lysinibacillus sphaericus". Environmental Microbiology Reports. 6 (6): 730–737. doi:10.1111/1758-2229.12196. PMID 25756126.
- Schulenburg, H., Kurz, C.L., Ewbank, J.J. (2004). "Evolution of the innate immune system: the worm perspective". Immunological Reviews. 198: 36–58. doi:10.1111/j.0105-2896.2004.0125.x. PMID 15199953. S2CID 21541043.CS1 maint: multiple names: authors list (link)
- Hodgkin J, Partridge FA (2008). "Caenorhabditis elegans Meets Microsporidia: The Nematode Killers from Paris. PLoS Biol". PLOS Biology. 6 (12): 2634–7. doi:10.1371/journal.pbio.1000005. PMC 2605933. PMID 19108611.
- Ewbank, Jonathan (2006). "Signaling in the immune response". WormBook: 1–12. doi:10.1895/wormbook.1.83.1. PMC 4781569. PMID 18050470.
- Schulenburg, H., Ewbank, J.J (2007). "the genetics of pathogen avoidance in Caenorhabditis elegans". Molecular Microbiology. 66 (3): 563–570. doi:10.1111/j.1365-2958.2007.05946.x. PMID 17877707. S2CID 20783253.CS1 maint: multiple names: authors list (link)
- Luallen, Robert; Reinke, Aaron; Tong, Linda; Botts, Michael; Felix, Marie-Anne; Troemel, Emily (2016). "Discovery of a Natural Microsporidian Pathogen with a Broad Tissue Tropism in Caenorhabditis elegans". PLOS Pathogens. 12 (6): e1005724. bioRxiv 10.1101/047720. doi:10.1371/journal.ppat.1005724. PMC 4928854. PMID 27362540.
- Barron GL. "Nematophagous destroying fungi. Topics in Mycobiology [serial on the Internet] 1977; 1". Cite journal requires
|journal=
(help) - Jansson HB. (1994). "Adhesion of conidia of Drechmeria coniospora to Caenorhabditis elegans wild type and mutants". J Nematol. 26 (4): 430‐5. PMC 2619527. PMID 19279912.
- Félix MA, Ashe A, Piffaretti J, Wu G, Nuez I (2011). "Natural and Experimental Infection of Caenorhabditis Nematodes by Novel Viruses Related to Nodaviruses". PLOS Biol. 9 (1): e1000586. doi:10.1371/journal.pbio.1000586. PMC 3026760. PMID 21283608.
- Gammon, Don B. (2017-12-01). "Caenorhabditis elegans as an Emerging Model for Virus-Host Interactions". Journal of Virology. 91 (23). doi:10.1128/JVI.00509-17. ISSN 0022-538X. PMC 5686719. PMID 28931683.
- Hodgkin, J., Félix, MA., Clark, L.C., Stroud, D., Gravato-Nobre, M.J. (2013). "Two Leucobacter Strains Exert Complementary Virulence on Caenorhabditis Including Death by Worm-Star Formation". Curr. Biol. 23 (21): 2157–2161. doi:10.1016/j.cub.2013.08.060. PMC 3898767. PMID 24206844.CS1 maint: multiple names: authors list (link)
- Höfte, H., Whiteley, H.R. (1989). "Insecticidal crystal proteins of Bacillus thuringiensis". Microbiol. Rev. 53 (2): 242–255. doi:10.1128/MMBR.53.2.242-255.1989. PMC 372730. PMID 2666844.CS1 maint: multiple names: authors list (link)
- Schulenburg, H., Müller S. (2004). "Natural variation in the response of Caenorhabditis elegans towards Bacillus thuringiensis". Parasitology. 128 (4): 433–443. doi:10.1017/s003118200300461x. PMID 15151149.CS1 maint: multiple names: authors list (link)
- Wei J.Z.; Hale K.; Carta L.; Platzer E.; Wong C.; Fang S-C.; Aroian R.V. (2003). "Bacillus thuringiensis crystal proteins that target nematodes". PNAS. 100 (5): 2760–2765. Bibcode:2003PNAS..100.2760W. doi:10.1073/pnas.0538072100. PMC 151414. PMID 12598644.
- Borgonie G.; Van Driessche R.; Leyns F.; Arnaut G.; De Waele D.; Coomans A (1995). "Germination of Bacillus thuringiensis Spores in Bacteriophagous Nematodes (Nematoda: Rhabditida)". Journal of Invertebrate Pathology. 65 (1): 61–67. doi:10.1006/jipa.1995.1008. PMID 7876593.
- Salamitou S.; Ramisse F.; Brehélin M.; Bourget D.; Gilois N.; Gominet M.; Hernandez E.; Lereclus D. (2000). "the plcR regulon is involved in the opportunistic properties of Bacillus thuringiensis and Bacillus cereus in mice and insects". Microbiology. 146 (11): 2825–2832. doi:10.1099/00221287-146-11-2825. PMID 11065361.
Further reading
- Frézal, L., Félix, MA., C. elegans outside the Petri dish (http://lens.elifesciences.org/05849/)
- Cabreiro F, Gems D (2013). "Worms need microbes too: microbiota, health and aging in Caenorhabditis elegans". EMBO Mol Med. 5 (9): 1300–10. doi:10.1002/emmm.201100972. PMC 3799487. PMID 23913848.
- Petersen C., Dirksen P., Schulenburg H., Why we need more ecology for genetic models like C. elegans(http://www.cell.com/trends/genetics/abstract/S0168-9525%2814%2900198-X)