Thermal conductivity measurement
There are a number of possible ways to measure thermal conductivity, each of them suitable for a limited range of materials, depending on the thermal properties and the medium temperature. Three classes of methods exist to measure the thermal conductivity of a sample: steady-state, time-domain, and frequency-domain methods.
Steady-state methods
In general, steady-state techniques perform a measurement when the temperature of the material measured does not change with time. This makes the signal analysis straightforward (steady state implies constant signals). The disadvantage is that a well-engineered experimental setup is usually needed.
Steady-state methods, in general, work by applying a known heat flux, , to a sample with a surface area, , and thickness, ; once the sample's steady-state temperature is reached, the difference in temperature, , across the thickness of the sample is measured. After assuming one-dimensional heat flow and an isotropic medium, Fourier's Law is then used to calculate the measured thermal conductivity, :
Major sources of error in steady-state measurements include radiative and convective heat losses in the setup, as well as errors in the thickness of the sample propagating to the thermal conductivity.
In geology and geophysics, the most common method for consolidated rock samples is the divided bar. There are various modifications to these devices depending on the temperatures and pressures needed as well as sample sizes. A sample of unknown conductivity is placed between two samples of known conductivity (usually brass plates). The setup is usually vertical with the hot brass plate at the top, the sample in between then the cold brass plate at the bottom. Heat is supplied at the top and made to move downwards to stop any convection within the sample. Measurements are taken after the sample has reached to the steady state (with zero heat gradient or constant heat over entire sample), this usually takes about 30 minutes and over.
Other steady-state methods
For good conductors of heat, Searle's bar method can be used.[1] For poor conductors of heat, Lees' disc method can be used.[2]
Time-domain methods
The transient techniques perform a measurement during the process of heating up. The advantage is that measurements can be made relatively quickly. Transient methods are usually carried out by needle probes.
Non-steady-state methods to measure the thermal conductivity do not require the signal to obtain a constant value. Instead, the signal is studied as a function of time. The advantage of these methods is that they can in general be performed more quickly, since there is no need to wait for a steady-state situation. The disadvantage is that the mathematical analysis of the data is in general more difficult.
Transient hot wire method
The transient hot wire method (THW) is a very popular, accurate and precise technique to measure the thermal conductivity of gases, liquids,[3] solids,[4] nanofluids[5] and refrigerants[6] in a wide temperature and pressure range. The technique is based on recording the transient temperature rise of a thin vertical metal wire with infinite length when a step voltage is applied to it. The wire is immersed in a fluid and can act both as an electrical heating element and a resistance thermometer. The transient hot wire method has advantage over the other thermal conductivity method since there is a fully developed theory and there is no calibration or single-point calibration. Furthermore because of the very small measuring time (1 s) there is no convection present in the measurements and only the thermal conductivity of the fluid is measured with very high accuracy.
The most of the THW sensors used in academia consist of two identical very thin wires with only difference in the length.[3] Sensors using a single wire,[7][8] are used both in academia and industry with the advantage over the two-wire sensors the ease of handling of the sensor and change of the wire.
An ASTM standard is published for the measurements of engine coolants using a single-transient hot wire method.[9]
Transient plane source method
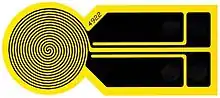
Transient Plane Source Method, utilizing a plane sensor and a special mathematical model describing the heat conductivity, combined with electronics, enables the method to be used to measure Thermal Transport Properties. It covers a thermal conductivity range of at least 0.01-500 W/m/K (in accordance with ISO 22007-2) and can be used for measuring various kinds of materials, such as solids, liquid, paste and thin films etc. In 2008 it was approved as an ISO-standard for measuring thermal transport properties of polymers (November 2008). This TPS standard also covers the use of this method to test both isotropic and anisotropic materials.
The Transient Plane Source technique typically employs two samples halves, in-between which the sensor is sandwiched. Normally the samples should be homogeneous, but extended use of transient plane source testing of heterogeneous material is possible, with proper selection of sensor size to maximize sample penetration. This method can also be used in a single-sided configuration, with the introduction of a known insulation material used as sensor support.
The flat sensor consists of a continuous double spiral of electrically conducting nickel (Ni) metal, etched out of a thin foil. The nickel spiral is situated between two layers of thin polyimide film Kapton. The thin Kapton films provides electrical insulation and mechanical stability to the sensor. The sensor is placed between two halves of the sample to be measured. During the measurement a constant electrical effect passes through the conducting spiral, increasing the sensor temperature. The heat generated dissipates into the sample on both sides of the sensor, at a rate depending on the thermal transport properties of the material. By recording temperature vs. time response in the sensor, the thermal conductivity, thermal diffusivity and specific heat capacity of the material can be calculated. For highly conducting materials, very large samples are needed (some litres of volume).
Modified transient plane source (MTPS) method
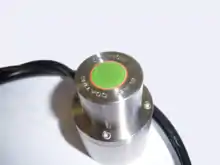
A variation of the above method is the Modified Transient Plane Source Method (MTPS) developed by Dr. Nancy Mathis. The device uses a one-sided, interfacial, heat reflectance sensor that applies a momentary, constant heat source to the sample. The difference between this method and traditional transient plane source technique described above is that the heating element is supported on a backing, which provides mechanical support, electrical insulation and thermal insulation. This modification provides a one-sided interfacial measurement in offering maximum flexibility in testing liquids, powders, pastes and solids.
Transient line source method
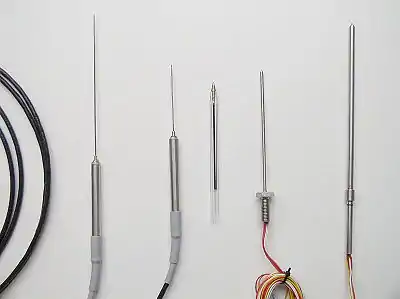
The physical model behind this method is the infinite line source with constant power per unit length. The temperature profile at a distance at time is as follows
where
- is the power per unit length, in [W·m−1]
- is the thermal conductivity of the sample, in [W·m−1·K−1]
- is the exponential integral, a transcendent mathematical function
- is the radial distance to the line source
- is the thermal diffusivity, in [m2·s−1]
- is the amount of time that has passed since heating has started, in [s]
When performing an experiment, one measures the temperature at a point at fixed distance, and follows that temperature in time. For large times, the exponential integral can be approximated by making use of the following relation
where
- is the Euler gamma constant
This leads to the following expression
Note that the first two terms in the brackets on the RHS are constants. Thus if the probe temperature is plotted versus the natural logarithm of time, the thermal conductivity can be determined from the slope given knowledge of Q. Typically this means ignoring the first 60 to 120 seconds of data and measuring for 600 to 1200 seconds. Typically, this method is used for gases and liquids whose thermal conductivities are between 0.1 and 50 W/(mK). If the thermal conductivities are too high, the diagram often does not show a linearity, so that no evaluation is possible.[10]
Modified transient line source method
A variation on the Transient Line Source method is used for measuring the thermal conductivity of a large mass of the earth for Geothermal Heat Pump (GHP/GSHP) system design. This is generally called Ground Thermal Response Testing (TRT) by the GHP industry.[11][12][13] Understanding the ground conductivity and thermal capacity is essential to proper GHP design, and using TRT to measure these properties was first presented in 1983 (Mogensen). The now commonly used procedure, introduced by Eklöf and Gehlin in 1996 and now approved by ASHRAE involves inserting a pipe loop deep into the ground (in a well bore, filling the anulus of the bore with a grout substance of known thermal properties, heating the fluid in the pipe loop, and measuring the temperature drop in the loop from the inlet and return pipes in the bore. The ground thermal conductivity is estimated using the line source approximation method—plotting a straight line on the log of the thermal response measured. A very stable thermal source and pumping circuit are required for this procedure.
More advanced Ground TRT methods are currently under development. The DOE is now validating a new Advanced Thermal Conductivity test said to require half the time as the existing approach, while also eliminating the requirement for a stable thermal source.[14] This new technique is based on multi-dimensional model-based TRT data analysis.
Laser flash method
The laser flash method is used to measure thermal diffusivity of a thin disc in the thickness direction. This method is based upon the measurement of the temperature rise at the rear face of the thin-disc specimen produced by a short energy pulse on the front face. With a reference sample specific heat can be achieved and with known density the thermal conductivity results as follows
where
- is the thermal conductivity of the sample, in [W·m−1·K−1]
- is the thermal diffusivity of the sample, in [m2 ·s−1]
- is the specific heat capacity of the sample, in [J·kg−1·K−1]
- is the density of the sample, in [kg·m−3]
It is suitable for a multiplicity of different materials over a broad temperature range (−120 °C to 2800 °C).[15]
Time-domain thermoreflectance method
Time-domain thermoreflectance is a method by which the thermal properties of a material can be measured, most importantly thermal conductivity. This method can be applied most notably to thin film materials, which have properties that vary greatly when compared to the same materials in bulk. The idea behind this technique is that once a material is heated up, the change in the reflectance of the surface can be utilized to derive the thermal properties. The change in reflectivity is measured with respect to time, and the data received can be matched to a model which contain coefficients that correspond to thermal properties.
DynTIM method
DynTIM is a bulk thermal conductivity measurement system. DynTIM works by imitating the environmental parameters of real thermal interface materials, using a power diode for a heater or temperature sensor element.[16] By having strong thermal insulation surrounding the diode, heat escapes only through an exposed cooling tab, which is used as the probe for the thermal interface material measurements. This method shares similarities with the ASTM D5470 standard, such as the measurement of the thermal resistance at different material thickness levels.[17] The system is designed to measure high thermal conductivity thermal interface materials. Its applicability for the measurement of insulators is more limited.
Frequency-domain methods
3ω-method
One popular technique for electro-thermal characterization of materials is the 3ω-method, in which a thin metal structure (generally a wire or a film) is deposited on the sample to function as a resistive heater and a resistance temperature detector (RTD). The heater is driven with AC current at frequency ω, which induces periodic joule heating at frequency 2ω due to the oscillation of the AC signal during a single period. There will be some delay between the heating of the sample and the temperature response which is dependent upon the thermal properties of the sensor/sample. This temperature response is measured by logging the amplitude and phase delay of the AC voltage signal from the heater across a range of frequencies (generally accomplished using a lock-in-amplifier). Note, the phase delay of the signal is the lag between the heating signal and the temperature response. The measured voltage will contain both the fundamental and third harmonic components (ω and 3ω respectively), because the Joule heating of the metal structure induces oscillations in its resistance with frequency 2ω due to the temperature coefficient of resistance (TCR) of the metal heater/sensor as stated in the following equation:
- ,
where C0 is constant. Thermal conductivity is determined by the linear slope of ΔT vs. log(ω) curve. The main advantages of the 3ω-method are minimization of radiation effects and easier acquisition of the temperature dependence of the thermal conductivity than in the steady-state techniques. Although some expertise in thin film patterning and microlithography is required, this technique is considered as the best pseudo-contact method available.[18] (ch23)
Frequency-domain hot-wire method
The transient hot wire method can be combined with the 3ω-method to accurately measure the thermal conductivity of solid and molten compounds from room temperature up to 800 °C. In high temperature liquids, errors from convection and radiation make steady-state and time-domain thermal conductivity measurements vary widely;[19] this is evident in the previous measurements for molten nitrates.[20] By operating in the frequency-domain, the thermal conductivity of the liquid can be measured using a 25 μm diameter hot-wire while rejecting the influence of ambient temperature fluctuations, minimizing error from radiation, and minimizing errors from convection by keeping the probed volume below 1 μL.[21]
Freestanding sensor-based 3ω-method
The freestanding sensor-based 3ω technique[22][23] is proposed and developed as a candidate for the conventional 3ω method for thermophysical properties measurement. The method covers the determination of solids, powders and fluids from cryogenic temperatures to around 400 K.[24] For solid samples, the method is applicable to both bulks and tens of micrometers thick wafers/membranes,[25] dense or porous surfaces.[26] The thermal conductivity and thermal effusivity can be measured using selected sensors, respectively. Two basic forms are now available: the linear source freestanding sensor and the planar source freestanding sensor. The range of thermophysical properties can be covered by different forms of the technique, with the exception that the recommended thermal conductivity range where the highest precision can be attained is 0.01 to 150 W/m•K for the linear source freestanding sensor and 500 to 8000 J/m2•K•s0.5 for the planar source freestanding sensor.
Measuring devices
A thermal conductance tester, one of the instruments of gemology, determines if gems are genuine diamonds using diamond's uniquely high thermal conductivity.
For an example, see Measuring Instrument of Heat Conductivity of ITP-MG4 "Zond" (Russia).[27]
Standards
- EN 12667, "Thermal performance of building materials and products. Determination of thermal resistance by means of guarded hot plate and heat flow meter methods. Products of high and medium thermal resistance", ISBN 0-580-36512-3.
- ISO 8301, "Thermal insulation – Determination of steady-state thermal resistance and related properties – Heat flow meter apparatus"
- ISO 8497, "Thermal insulation – Determination of steady-state thermal transmission properties of thermal insulation for circular pipes", ISBN 0-580-26907-8
- ISO 22007-2:2008 "Plastics – Determination of thermal conductivity and thermal diffusivity – Part 2: Transient plane heat source (hot disc) method"
- ISO 22007-4:2008 "Plastics – Determination of thermal conductivity and thermal diffusivity – Part 4: Laser flash method"[15]
- IEEE Standard 442–1981, "IEEE guide for soil thermal resistivity measurements", ISBN 0-7381-0794-8. See also soil thermal properties. [28]
- IEEE Standard 98-2002, "Standard for the Preparation of Test Procedures for the Thermal Evaluation of Solid Electrical Insulating Materials", ISBN 0-7381-3277-2 [29]
- ASTM Standard C518 – 10, "Standard Test Method for Steady-State Thermal Transmission Properties by Means of the Heat Flow Meter Apparatus"
- ASTM Standard D5334-14, "Standard Test Method for Determination of Thermal Conductivity of Soil and Soft Rock by Thermal Needle Probe Procedure"
- ASTM Standard D5470-06, "Standard Test Method for Thermal Transmission Properties of Thermally Conductive Electrical Insulation Materials"
- ASTM Standard E1225-04, "Standard Test Method for Thermal Conductivity of Solids by Means of the Guarded-Comparative-Longitudinal Heat Flow Technique"
- ASTM Standard D5930-01, "Standard Test Method for Thermal Conductivity of Plastics by Means of a Transient Line-Source Technique"
- ASTM Standard D2717-95, "Standard Test Method for Thermal Conductivity of Liquids"
References
- Searle's Bar for a good heat conductor. Media.uws.ac.uk. Retrieved on 2017-09-05.
- Ian Hickson's Lees' Disc Experiment. Academia.hixie.ch. Retrieved on 2013-12-12.
- Wakeham, W.A.; Nagashima, A.; Sengers, J.V., eds. (1991). "Measurement of the Transport Properties of Fluids". Experimental Thermodynamics, Volume III. Oxford: Blackwell Scientific Publications.
- Assael, M.J.; Antoniadis, K.D.; Metaxa, I.N.; Mylona, S.K.; Assael, J.-A.M.; Wu, J.; Hu, M. (2015). "A Novel Portable Absolute Transient Hot-Wire Instrument for the Measurement of the Thermal Conductivity of Solids". International Journal of Thermophysics. 36 (10–11): 3083–3105. Bibcode:2015IJT....36.3083A. doi:10.1007/s10765-015-1964-6. S2CID 118547999.
- Assael, M.J.; Chen, C.F.; Metaxa, I.; Wakeham, W.A. (2004). "Thermal conductivity of suspensions of carbon nanotubes in water". International Journal of Thermophysics. 25 (4): 971–985. Bibcode:2004IJT....25..971A. doi:10.1023/B:IJOT.0000038494.22494.04. S2CID 97459543.
- Mylona, Sofia K.; Hughes, Thomas J.; Saeed, Amina A.; Rowland, Darren; Park, Juwoon; Tsuji, Tomoya; Tanaka, Yukio; Seiki, Yoshio; May, Eric F. (2019). "Thermal conductivity data for refrigerant mixtures containing R1234yf and R1234ze(E)". The Journal of Chemical Thermodynamics. 133: 135–142. doi:10.1016/j.jct.2019.01.028.
- Nagasaka, N.; Nagashima, A. (1981). "Simultaneous measurement of the thermal conductivity and the thermal diffusivity of liquids by the transient hot‐wire method". Review of Scientific Instruments. 52 (2): 229–232. Bibcode:1981RScI...52..229N. doi:10.1063/1.1136577.
- Fujii, M.; Zhang, X.; Imaishi, N.; Fujiwara, S.; Sakamoto, T. (1997). "Simultaneous measurements of thermal conductivity and thermal diffusivity of liquids under microgravity conditions". International Journal of Thermophysics. 18 (2): 327–339. Bibcode:1997IJT....18..327F. doi:10.1007/BF02575164. S2CID 122155913.
- ASTM D7896-14 – Standard Test Method for Thermal Conductivity, Thermal Diffusivity and Volumetric Heat Capacity of Engine Coolants and Related Fluids by Transient Hot Wire Liquid Thermal Conductivity Method, ASTM International, West Conshohocken, PA, 2014, doi:10.1520/D7896-14
- tec-science (2020-02-10). "Transient-Hot-Wire method method for determining thermal conductivity (THW)". tec-science. Retrieved 2020-02-10.
- Chiasson, A.D. (1999). "Advances in modeling of ground source heat pump systems" (PDF). Oklahoma State University. Retrieved 2009-04-23. Cite journal requires
|journal=
(help) - http://www.igshpa.okstate.edu/research/papers/tc_testing_copyright.pdf Soil Thermal Conductivity Tests Richard A. Beier, Mechanical Engineering Technology Department, Oklahoma State University
- http://www.hvac.okstate.edu/sites/default/files/pubs/papers/2002/08-Witte_VanGelder_Spitler_02.pdf In Situ Measurement of Ground Thermal Conductivity: A Dutch Perspective, Henk J.L. Witte, Guus J. van Gelder, Jeffrey D. Spitler
- http://www.sbv.org/a/pages/level3-geothermal-round1-2 Advanced Thermal Conductivity Testing for Geothermal Heating and Cooling Systems, U.S. Department of Energy, Small Business Vouchers Pilot
- ISO22007-4:2008 Plastics – Determination of thermal conductivity and thermal diffusivity – Part 4: Laser flash method
- Vass-Várnai, András; Sárkány, Zoltán; Rencz, Márta (September 2012). "Characterization method for thermal interface materials imitating an in-situ environment". Microelectronics Journal. 43 (9): 661–668. doi:10.1016/j.mejo.2011.06.013.
- A. Vass-Várnai, M.Rencz: ‘Testing interface thermal resistance‘ In: Proceedings of eTherm'08 - The 1st International Symposium on Thermal Design and Thermophysical Property for Electronics. Tsukuba, Japan, 2008.06.18-2008.06.20. pp. 73-76.
- Rowe, David Michael. Thermoelectrics handbook : macro to nano / edited by D.M. Rowe. Boca Raton: CRC/Taylor & Francis, 2006. ISBN 0-8493-2264-2
- Chliatzou, Ch. D.; Assael, M. J.; Antoniadis, K. D.; Huber, M. L.; Wakeham, W. A. (2018). "Reference Correlations for the Thermal Conductivity of 13 Inorganic Molten Salts". Journal of Physical and Chemical Reference Data. 47 (3): 033104. Bibcode:2018JPCRD..47c3104C. doi:10.1063/1.5052343. ISSN 0047-2689. PMC 6459620. PMID 30983644.
- Zhao, Qing-Guo; Hu, Chun-Xu; Liu, Su-Jie; Guo, Hang; Wu, Yu-Ting (2017-12-01). "The thermal conductivity of molten NaNO3, KNO3, and their mixtures". Energy Procedia. Leveraging Energy Technologies and Policy Options for Low Carbon Cities. 143: 774–779. doi:10.1016/j.egypro.2017.12.761. ISSN 1876-6102.
- Wingert, M. C.; Zhao, A. Z.; Kodera, Y.; Obrey, S. J.; Garay, J. E. (2020-05-01). "Frequency-domain hot-wire sensor and 3D model for thermal conductivity measurements of reactive and corrosive materials at high temperatures". Review of Scientific Instruments. 91 (5): 054904. Bibcode:2020RScI...91e4904W. doi:10.1063/1.5138915. ISSN 0034-6748.
- Qiu, L.; Tang, D. W.; Zheng, X. H.; Su, G. P. (2011). "The freestanding sensor-based 3ω technique for measuring thermal conductivity of solids: Principle and examination". Review of Scientific Instruments. 82 (4): 045106–045106–6. Bibcode:2011RScI...82d5106Q. doi:10.1063/1.3579495. PMID 21529038.
- Qiu, L.; Zheng, X. H.; Zhu, J.; Tang, D. W. (2011). "Note: Non-destructive measurement of thermal effusivity of a solid and liquid using a freestanding serpentine sensor-based 3ω technique". Review of Scientific Instruments. 82 (8): 086110–086110–3. Bibcode:2011RScI...82h6110Q. doi:10.1063/1.3626937. PMID 21895288.
- Qiu, L.; Zheng, X. H.; Su, G. P.; Tang, D. W. (21 September 2011). "Design and Application of a Freestanding Sensor Based on 3ω Technique for Thermal-Conductivity Measurement of Solids, Liquids, and Nanopowders". International Journal of Thermophysics. 34 (12): 2261–2275. doi:10.1007/s10765-011-1075-y. S2CID 121187257.
- Qiu, L.; Zheng, X.H.; Yue, P.; Zhu, J.; Tang, D.W.; Dong, Y.J.; Peng, Y.L. (March 2015). "Adaptable thermal conductivity characterization of microporous membranes based on freestanding sensor-based 3ω technique". International Journal of Thermal Sciences. 89: 185–192. doi:10.1016/j.ijthermalsci.2014.11.005.
- Qiu, L.; Li, Y. M.; Zheng, X. H.; Zhu, J.; Tang, D. W.; Wu, J. Q.; Xu, C. H. (1 December 2013). "Thermal-Conductivity Studies of Macro-porous Polymer-Derived SiOC Ceramics". International Journal of Thermophysics. 35 (1): 76–89. doi:10.1007/s10765-013-1542-8. S2CID 95284477.
- "Измеритель теплопроводности ИТП-МГ4 "Зонд"". www.stroypribor.ru. Retrieved 2018-07-14.
- IEEE Guide for Soil Thermal Resistivity Measurements. 1981. doi:10.1109/IEEESTD.1981.81018. ISBN 978-0-7381-0794-3.
- IEEE Standard for the Preparation of Test Procedures for the Thermal Evaluation of Solid Electrical Insulating Materials. 2002. doi:10.1109/IEEESTD.2002.93617. ISBN 0-7381-3277-2.
External links
- An alternative traditional method using real thermometers is described at .
- A brief review of new methods measuring thermal conductivity, thermal diffusivity and specific heat within a single measurement is available at .
- A brief description of Modified Transient Plane Source (MTPS) at http://patents.ic.gc.ca/opic-cipo/cpd/eng/patent/2397102/page/2397102_20120528_description.pdf