Hydrothermal mineral deposit
Hydrothermal mineral deposits are accumulations of valuable minerals which formed from hot waters circulating in Earth's crust through fractures. They eventually create rich-metallic fluids concentrated in a selected volume of rock, which become supersaturated and then precipitate ore minerals. In some occurrences, minerals can be extracted at a profit by mining. Discovery of mineral deposits consumes considerable time and resources and only about one in every one thousand prospects explored by companies are eventually developed into a mine.[1] A mineral deposit is any geologically significant concentration of an economically useful rock or mineral present in a specified area.[2] The presence of a known but unexploited mineral deposit implies a lack of evidence for profitable extraction.[2]
Hydrothermal mineral deposits are divided into six main subcategories: porphyry, skarn, volcanogenic massive sulfide (VMS), sedimentary exhalative (SEDEX), and epithermal and Mississippi Valley-type (MVT) deposits. Each hydrothermal mineral deposit has different distinct structures, ages, sizes, grades, geological formation, characteristics and, most importantly, value.[3] Their names derive from their formation, geographical location or distinctive features.[3]
Generally, porphyry-type mineral deposits form in hydrothermal fluid circulation systems developed around felsic to intermediate magma chambers and/or cooling plutons. However, they did not precipitate directly from the magma. While, a skarn deposit is an assemblage of ore and calc-silicate minerals, formed by metasomatic replacement of carbonate rocks in the contact aureole of a pluton.[4] Volcanogenic massive sulfide deposits form when mafic magma at depth, (perhaps a few kilometers beneath the surface), acts as a heat source, causing convective circulation of seawater through the oceanic crust. The hydrothermal fluid leaches metals as it descends and precipitates minerals as it rises. Sedimentary exhalative deposits, also called sedex deposits, are lead-zinc sulfide deposits formed in intracratonic sedimentary basins by the submarine venting of hydrothermal fluids. These deposits are typically hosted in shale. Hydrothermal epithermal deposits consist of geological veins or groups of closely spaced geological veins. Finally, Mississippi Valley-type (MVT) are hosted in limestone or dolomite that was deposited in a shallow marine environment in a tectonically stable intraplate environment. As expected in such an environment, volcanic rocks, folding and regional metamorphism are absent as a general rule. MVT deposits commonly lie in close proximity to evaporites.[5]
Background
A mineral ore deposit is the volume of rock that can be mined at a profit.[6] Therefore, there are many variants that can define whether a mineral deposit is profitable or not, such as price, tonnage, or location. Mineral commodities can be classified as metals or non-metals.[2] Metals refer to elements of the periodic table which include base, ferrous, minor fissionable and precious metals. On the other hand, non-metals refer to industrial minerals such as gypsum, diamonds, oil, coal and aggregate. Hydrothermal deposits of economically valuable and recoverable minerals are generally considered scarce, meaning such deposits are very small relative to the total area of earth's surface.[2]
Hydrothermal mineral deposits play a key role in nearly all modern industrial activities.
Igneous | Ore minerals are precipitated directly from a magma. |
---|---|
Sedimentary | Ore minerals are concentrated or formed by sedimentary processes. |
Metamorphic | Ore minerals are formed during metamorphism. |
Hydrothermal | Ore minerals are precipitated by a hydrothermal solution percolating through intergranular spaces and along bedding planes and fractures in the host rocks. |
According to some authors, the hydrothermal solutions can have four origins, although any single volume of hydrothermal solution is commonly a mixture of two or more types:[8]
- Deuteric fluid derived from magma at a late stage of crystallization[8]
- Metamorphic fluid derived by progressive removal of hydrothermal fluids during regional metamorphism[8]
- Meteoric water descending from the surface[8]
- Fluid formed by the degassing of the core? and mantle[8]
- Basinal brines are also considered as a possible source of hydrothermal fluids. The fluids are thought to be connate water expelled from sediments with compactions and tectonic forces.
Ore minerals can form at the same time and from the same processes as the host rock, also termed as syngenetic, they can form slightly after the formation of the host rock, perhaps during weathering or compaction, also termed as diagenetic, or they can form much later than the host rock or epigenetic.[9] Host rock is the rock surrounding the ore deposit.[10][5]
Hydrothermal deposit Subcategory | Known abbreviation | Formation | Principal metals | Host rocks |
---|---|---|---|---|
Porphyry | — | Epigenetic | Cu, Mo, Au | The ore is spatially associated with one or more high-level intrusions of felsic to intermediate composition such as granite, granodiorite or diorite.[11] |
Skarn | — | Epigenetic | Cu, Mo, Ag, Au | A skarn deposit is an assemblage of ore and calc-silicate minerals, formed by metasomatic replacement of carbonate rocks in the contact aureole of a pluton.[12] |
Volcanogenic massive sulphide | VMS | Syngenetic | Cu, Zn, Pb | The host rocks are mainly volcanic, with the felsic volcanic rocks pointing to a convergent setting (island arc or orogenic belt).[13] |
Sedimentary exhalative | SEDEX | Syngenetic | Zn, Pb | These deposits are commonly stratiform and are typically hosted in shale.[3] |
Epithermal | — | Epigenetic | Au, Ag | The host rocks can be sheared muscovite granite, small plutons. |
Mississippi Valley-type | MVT | Epigenetic | Pb, Zn | MVT deposits are hosted in carbonate rocks, whereas sedex deposits are found within marine shales |
Porphyry Ore Deposits

Porphyry deposits account for most of the copper and molybdenum world production, 60 and 95 percent of its supply respectively.[1]
Porphyry-type ore deposits form in hydrothermal fluid circulation systems developed above and around high-level, subvolcanic felsic to intermediate magma chambers and/or cooling plutons. The ore is temporally and genetically related to the intrusions, but did not precipitate directly from the magma.[1]
Formation
Porphyry mineral deposits are formed when two plate tectonic plates collide in an advanced subduction zone, then cools off reacting with existing rocks and finally forming a copper deposit. The level of displacement is usually shallow at less than two kilometers below surface in an active volcanic area.
An example for a typical arc-island porphyry deposit is described as follows:[1]
- The formation starts during early volcanism on the seafloor above a subduction zone in an oceanic-oceanic collision zone[1]
- Then as the magma crystallizes, volatiles such as water, carbon dioxide and sulfur dioxide increase in concentration in the liquid phase of the magma.[1]
- Eventually, at a very late stage of crystallization, the volatile concentration becomes so great that a separate hydrothermal fluid phase separated from the silicate magma.[1]
- As the amount of hydrothermal fluid increases, vapour pressure increases.[1]
- At some point, vapour pressure exceeds the strength of the overlying roof rocks and a volcanic explosion takes place fracturing the overlying rock.[1]
- The sudden reduction in confining pressure on the remaining magma leads to instantaneous vigorous boiling of the magma as more and more volatiles separate.[1]
- Consequently, the closing of the fractures in the roof rocks by precipitation of minerals allow confining pressure to increase once again.[1]
- As time passed, increasingly felsic magmas rise up into the core of the volcano. Some of these later magmas probably erupt on the surface, forming new layers of volcanic rocks that will later be removed by erosion.[1]
Finally, volcanic activity ceased and erosion removed the upper portions of the volcano and exposed the intrusive rocks and stockwork mineralization that used to lie within.[14]
Porphyry characteristics
Age | Average age of 13 million years, continental and oceanic arcs of Tertiary and Quaternary age.[15] |
---|---|
Size | Amongst the largest in the world, especially porphyry-type deposits.[16] |
Location | The 25 biggest porphyry deposits are found in the southwest Pacific and South America.[17] |
Host rocks | Ore is associated with one or more subvolcanic intrusions of felsic to intermediate composition such as granite, granodiorite or diorite.[18] |
Economic metals | In island arc settings where the host plutons are typically andesitic in composition, the elements of economic interest are mainly copper and gold.
In contrast, those that occur in continental orogenic belts are typically rhyolitic in composition and carry copper, molybdenum and gold, and in some cases tin and/or tungsten.[18] |
Grade | Commonly low in grade and have relatively low dollar value[18] |
Fractures | Ore minerals are generally confined to small veinlets and less common larger veins that formed as fracture fillings in the host rocks.[18] Hydrothermal breccia can often occur, sometimes in the form of pebble dikes.[19] |
Hydrothermal alteration | The wallrock on both sides of each veinlet is typically altered to varying degrees.
The primary silicate minerals such as feldspar and amphibole are replaced by hydrothermal minerals stable at temperatures of about 400 °C or less such as chlorite, epidote, muscovite and quartz.[18] Alteration assemblages typically include proximal potassic, intermediate phyllic or QSP, and more distal propylitic and argillic alteration.[20] Where veinlets are close together, the zones of alteration around each veinlet overlap, making the whole rock hydrothermally altered.[21] |
Mining activity | Bingham Mine, The Chuquicamata deposit, El Teniente deposit, Henderson Mine |
Skarn Mineral Deposits
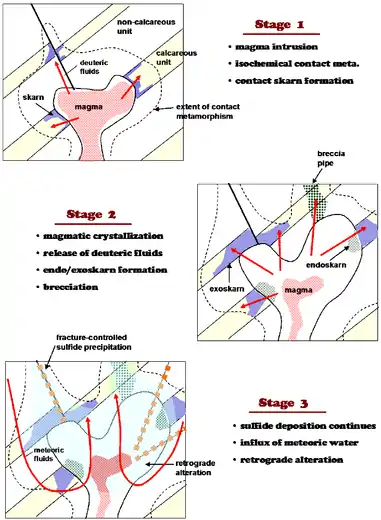
Skarn Mineral Deposits tend to be small in size but high in mineral grade. Therefore, it is a balance and challenge to find a profitable skarn orebody.
Geologically speaking, a skarn deposit is an assemblage of ore and calc-silicate minerals, formed by metasomatic replacement of carbonate rocks in the contact aureole of a pluton. Typical calc-silicate minerals are garnet, epidote, pyroxene, chlorite, amphibole and quartz – magnesian minerals dominate if dolomite is replaced whereas calcic minerals dominate where limestone is replaced.[22]
Skarn deposits are of economic interest, since they are the source of numerous metals as well as minerals of industrial application.[22]
Formation
Skarn formation, as illustrated in the figure on the right, can be explained in three stages:[23]
- Intrusion of a felsic to intermediate magma body rich in volatiles. Contact metamorphism and minor metasomatism, skarn formation, occurs in favorable locations.[23]
- Continued crystallization of the magma and widespread release of volatiles as a hydrothermal fluid which causes widespread skarn formation and localized brecciation.[23]
- Characterized by decreasing temperatures and hydrothermal activity, during which sulfide deposition occurs in veins and retrograde alteration is common.[23]
There is a very close spatial association with the granite, the skarn occurs only within marble which is known to be a very reactive rock type, and the skarn has a chemical composition that is unlike any known igneous or sedimentary rock type. Furthermore, various structures such as flexures in the contact or impermeable hornfels beds affected the distribution and ore grade of the skarn zones.[24]
Skarn characteristics
Size | Relatively small, they tend to be less than 10 million tonnes, although a few large ones exist such as the Mission mine in Arizona, 320 million tonnes.[25] |
---|---|
Economic Metals | Tungsten, tin, molybdenum, copper, iron, lead-zinc and gold ores.[25] |
Geologic Features | Nonfoliate rock textures created by contact metamorphism such as hornfels and marble[25] |
Level of Emplacement | Close proximity to a felsic to intermediate pluton of relatively large size. Therefore, shallow depths.[25] |
Grade | Ore zones may grade laterally into calcic or dolomitic marble.[25] |
Geometry | Equidimensional geometries are most common.
Many orebodies are elongate along structural weaknesses such as faults and bedding planes The largest and thickest orebodies tend to occur where carbonate beds lie immediately above gently inclined pluton contacts.[25] |
Mining activity | Grasberg and Ertsberg Mines are part of a single mining complex in the glacier-capped mountains of Irian Jaya, Indonesia.
Together, they comprise the single largest copper-gold mine in the world, with reserves of 2.8 billion tonnes grading 1.1% Cu and 1.1 g/t Au.[26] |
Epithermal hydrothermal vein deposits
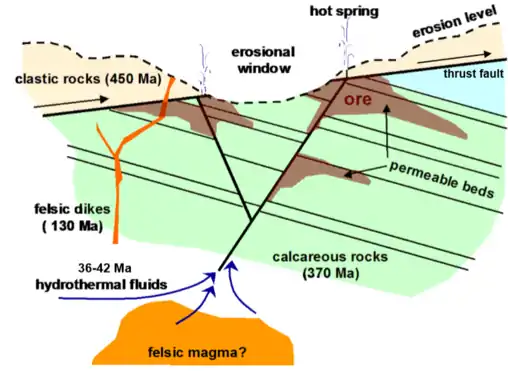
Hydrothermal vein ore deposits consist of discrete veins or groups of closely spaced veins. Veins are believed to be precipitated by hydrothermal solutions travelling along discontinuities in a rockmass.[7] They are commonly epithermal in origin, that is to say they form at relatively high crustal levels and moderate to low temperatures. They are epigenetic since they form after their host rocks.[7]
Formation
Hydrothermal vein deposits fall into three main categories:
- Felsic pluton association - many veins are spatially associated with felsic plutons, presumably because a pluton is a source of deuteric fluids.
- Mafic volcanic rock association - many veins and vein packages occur within mafic volcanic sequences such as the greenstone belts of the Canadian Shield.
- The metasedimentary association.
There are two main possibilities for the origin of the ore, both of which are hydrothermal:[27]
One possibility, the rise of a small body of felsic magma may have led to either the:
- Release of deuteric hydrothermal fluid, or
- The creation of a convective meteoric water system driven by the hot pluton.
Elements were leached from the already solidified portions of the pluton. The fluids would have migrated upward and outward, following fractures in the solidified part of the granite pluton, precipitating ore minerals in veins and altering the wallrocks.
The other possibility, a regional shearing event developed in the crust. Shearing take place at temperatures on the order of 300–400 °C. Thus, the shearing event may have been accompanied by the generation and movement of hydrothermal fluid as the crust was subjected to prograde devolatilization.[28] This fluid might have leached the ore elements from one part of the granite pluton and reprecipitated them in veins in another part of the same pluton, effectively concentrating them.[28]
Characteristics
Epithermal ore deposits form at shallow depth[29] and are typically tabular (two-dimensional) in geometry.[30]
Mining activity
Good examples are the gold-silver veins in northwestern Nevada and large ion veins such as the fluorspar veins in the St. Lawrence mine in Newfoundland[31] and the tin-bearing veins that made up the East Kemptville Mine in southwestern Nova Scotia.[32]
Volcanogenic massive sulfide mineral deposits
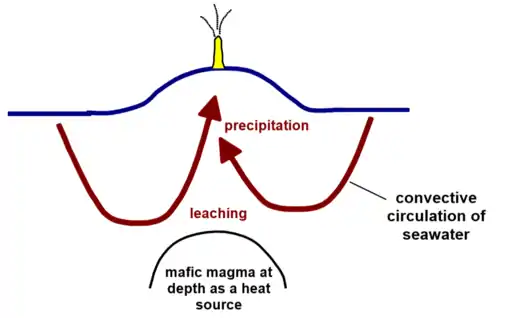
Volcanogenic massive sulfide (VMS) are responsible for almost a quarter of the world's zinc production while contributing for lead, silver and copper as well. VMS deposits tend to be of great size since they form over a long period of time and have a relatively high grade in valuable minerals. The main minerals in this deposit are sulphide minerals such as pyrite, sphalerite, chalcopyrite and galena.
The term “massive sulfide” deposit refers to any deposit containing more than 50% sulfide minerals. The modifier “volcanogenic” indicates that the massive sulfides are believed to be genetically related to volcanism that was ongoing at the time of sulfide deposition. Thus, VMS deposits are believed to be syngenetic or perhaps slightly diagenetic in age relative to their host volcanic rocks.
Formation
Deposition of VMS is due to mainly two reasons:[33]
- Mixing between ascending hot mineral-bearing fluids and the cold descending water.
- Cooling of the ascending high-temperature solution.
VMS deposits form in zones of extension and active volcanism. The original fluid is mainly cold, alkaline, deficient-in-metals sea water and in some cases it can include a lesser proportion of magmatic fluid.
The main source of the minerals comes from the volcanic rocks through which the sea water flows, taking with it the minerals of the volcanic rock.
The sea water is heated, convection currents are formed and they ascend carrying the minerals which are discharged at the bottom of the sea or immediately below the surface in the form of black smokers.[34]
Magma rises up from the mantle and then cools off in the crust and then releases volatile fluids that contain metals that are eventually transported up to the surface and over time these accumulations become mineral deposits.
As the high-temperature volatile fluids from the magma make contact with low-temperature liquids such as seawater that travel downwards via cracks and faults, producing, due to the large difference in temperature and chemical properties, mineral precipitation, yielding the black colour in the black smokers that end up showing up in the seafloor.
The host rocks are mainly volcanic, with the felsic volcanic rocks pointing to a convergent setting such as an island arc or orogenic belt. Minor sedimentary beds such as chert and slate are found in VMS deposits and they indicate marine deposition, below the wave base.
VMS deposits formed on the seafloor, in the same way that modern seafloor smokers are forming today. The most recent compilations of VMS deposits on land include about 1,100 deposits in more than 50 countries and 150 different mining camps or districts.[35]
VMS Characteristics
Age | Almost any age can potentially host a VMS deposit, the oldest VMS deposits are 3.4 billion years old.[36] |
---|---|
Size | Individual lenses that are a hundred meters thick and extend hundred meters along strike. The median deposit size is only about 70,000 tonnes.[33] |
Types | There are three of types of sulfide ore that can be found in these mineral deposits.[37]
|
Geometry | Typically tabular to lensoid, and range from less than 1 to more than 150 million tonnes. They often occur in clusters. |
Economic minerals | Chalcopyrite (Cu), sphalerite (Zn), galena (Pb), silver and gold. The dominant gangue minerals are quartz, pyrite and pyrrhotite.
Lenses of barite (BaSO4), gypsum or anhydrite are associated with the sulfides in some deposits.[36] |
Mining activity | Flin Flon, Manitoba, Canada, Kidd Creek Mine, Ontario, Canada |
Sedimentary Exhalative Mineral Deposits
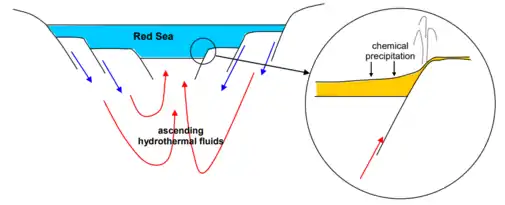
Sedimentary exhalative (SEDEX) deposits account for 40% of total world zinc production, 60% of lead and a significant proportion of silver. Despite their economic importance however, sedex deposits are relatively rare. A worldwide compilation of sedex deposits indicates that about 70 are known, of which 24 have been or are being mined. The majority is uneconomic to mine because of relatively low grade or unusually fine grain size, making mill recovery rather low.[38]
SEDEX deposits are lead-zinc sulfide deposits formed in intracratonic rift basins by the submarine venting of hydrothermal fluids. These deposits are commonly stratiform, tabular - lenticular and are typically hosted in shale however, sedimentary rocks detrictics or even carbonates could be the host.
Formation
SEDEX deposits form in sedimentary basins under a regional tectonic extensional environment, under the ocean where cold seawater (blue arrows) is mixed with basin water and through sinsedimentary faults flow towards the bottom of the basin, which are heated by the geothermal gradient, and later ascends by convective currents (red arrows).[39]
Model for the origin of the Red Sea sulfide deposits. Cold seawater (blue arrows) enters the seafloor via deep-seated fractures. As it descends, it heats up and leaches silicon, metals and other solutes from the seafloor basalts.
The source of sulfur can be by bacterial reduction of marine sulfate a process that takes place at the bottom of the basin. It can also come from the washing of the underlying series or by the thermochemical reduction of the marine sulfate. Precipitation of sulfide minerals could be triggered by inorganic precipitation and/or bacterial precipitation.
SEDEX Characteristics
Size | Average 41 million tonnes
Generally take the form of stratiform lenses with maximum thicknesses in the range of just 5 to 20 m.. In contrast, sphalerite tends to be concentrated in the lower grade outer portions of the ore bodies.[40] |
---|---|
Grade | 6.8% Zn, 3.5% Pb and 50 g/t Ag[40] |
Ore minerals | Zinc, lead, silver, copper, tin and tungsten[40] |
Geometry | Form of stratiform lenses with maximum thicknesses in the range of just 5 to 20 meters. |
Mining activity | Mt Isa, Australia, Red Dog, USA, Sullivan Mine |
Mississippi Valley type mineral deposits
Formation
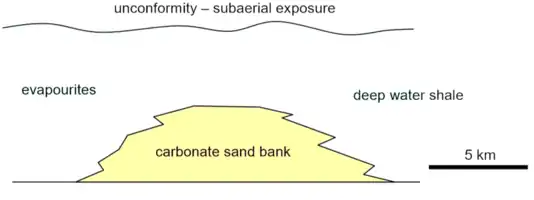
The deposits are hosted in limestone or dolomite that was deposited on shallow marine platforms in a tectonically stable intraplate environment. As expected in such an environment, volcanic rocks, folding and regional metamorphism are absent as a general rule. MVT deposits commonly lie in close proximity to evaporites and/or beneath unconformities.[40]
Deposits are discordant to bedding on a deposit scale, and are confined to specific stratigraphic horizons. Ore-hosting structures are most commonly zones of highly brecciated dolomite – these structures may be more or less vertical, crossing bedding at high angles, or they may be lensoid in shape extending in the same direction as bedding.
A petrogenetic model to explain MVT deposits in general:
- The ore minerals fill cavities and fractures in dolomite. Hence, they must be hydrothermal and epigenetic in origin.
- The hydrothermal fluids involved must have been fairly low in temperature since no rocks in the region are metamorphosed in any way.
- In addition, the presence of numerous cavities implies that the rocks were so shallow that confined pressure was insufficient to collapse the cavities.
- Moreover, the sphalerite is generally very pale yellow, meaning that it was a low temperature sphalerite rich in zinc and low in iron.
- Ore deposition occurred close to the surface, during or soon after karst development.
Deposits are discordant to bedding on a deposit scale.
Ore-hosting structures are most commonly zolinknes of highly brecciated dolomite.
These structures may be more or less vertical, crossing bedding at high angles, or they may be lensoid in shape extending in the same direction as bedding.
MVT Characteristics
Size | Tend to be less than 10 million tonnes each, and they tend to occur in clusters.
As many as 400 individual deposits occur within the upper Mississippi Valley mining district alone.[41] |
---|---|
Grade | Generally fall between 5% and 15% combined Pb plus Zn.
Iron sulfides are commonly minor, although pyrite and chalcopyrite can be present and are even abundant in a few deposits.[40] |
Host rocks | Limestones and dolomite, deposited on shallow marine platforms in a tectonically stable intraplate environment |
Ore minerals | Sphalerite and galena |
Mining activity | Pine Point Mine, NWT |
Mississippi Valley-type deposits can be compared with the Red Sea deposits, which are modern analogues of ancient sedex deposits, some differences can be made:[41]
- MVT deposits are hosted in carbonate rocks, whereas sedex deposits are found within marine shales
- MVT deposits are believed to form in very shallow water, most likely less than 50 meters in depth, whereas sedex deposits can form under relatively deep marine conditions
- Mineralization is characterized by coarse grain size, cavities, breccia fragments and euhedral crystals. In contrast, sedex mineralization is commonly fine grained and laminated
- MVT deposits are stratabound whereas sedex deposits tend to be stratiform
- Copper and pyrite/pyrrhotite are generally absent or minor in MVT deposits, whereas they can be more abundant in SEDEX deposits.
References
- Wilkinson, Jamie J. (2013-10-13). "Triggers for the formation of porphyry ore deposits in magmatic arcs" (PDF). Nature Geoscience. 6 (11): 917–925. Bibcode:2013NatGe...6..917W. doi:10.1038/ngeo1940. hdl:10044/1/52216. ISSN 1752-0894.
- Misra, Kula C. (2000), "Formation of Mineral Deposits", Understanding Mineral Deposits, Springer Netherlands, pp. 5–92, doi:10.1007/978-94-011-3925-0_2, ISBN 9789401057523
- Deb, Mihir; Sarkar, Sanjib Chandra (2017), "Energy Resources", Minerals and Allied Natural Resources and their Sustainable Development, Springer Singapore, pp. 351–419, doi:10.1007/978-981-10-4564-6_6, ISBN 9789811045639
- Peters, W.C. (1987-01-01). Exploration and mining geology. Second edition.
- Skinner, Brian J. (January 2005). "Introduction to Ore-Forming Processes". American Mineralogist. 90 (1): 276.1–276. doi:10.2138/am.2005.426. ISSN 0003-004X.
- M., Guilbert, John (2007). The geology of ore deposits. Waveland Pr. ISBN 978-1577664956. OCLC 918452788.
- Edwards, Richard; Atkinson, Keith (1986). Ore Deposit Geology and its Influence on Mineral Exploration. doi:10.1007/978-94-011-8056-6. ISBN 978-94-011-8058-0.
- Hedenquist, Jeffrey; Lowenstern, Jacob (1994-08-18). "The role of magmas in the formation of hydrothermal ore deposits". Nature. 370 (6490): 519–527. Bibcode:1994Natur.370..519H. doi:10.1038/370519a0.
- Ore Deposit Geology and its Influence on Mineral Exploration | Richard Edwards | Springer.
- C., Misra, Kula (2000). Understanding mineral deposits. Kluwer academic. ISBN 978-0045530090. OCLC 468703479.
- Robb, James (October 2005). "Editorial". Gait & Posture. 22 (2): 95. doi:10.1016/j.gaitpost.2005.07.009. ISSN 0966-6362.
- Guilbert, J. M. (1986). "BOOK REVIEWS". Geology. 14 (9): 816. Bibcode:1986Geo....14..816G. doi:10.1130/0091-7613(1986)14<816b:br>2.0.co;2. ISSN 0091-7613.
- Falcon, N. L.; Jensen, Mead L.; Bateman, Alan M.; Dixon, Colin J. (March 1981). "Economic Mineral Deposits". The Geographical Journal. 147 (1): 101. doi:10.2307/633431. ISSN 0016-7398. JSTOR 633431.
- Ulrich, T.; Günther, D.; Heinrich, C. A. (June 1999). "Gold concentrations of magmatic brines and the metal budget of porphyry copper deposits". Nature. 399 (6737): 676–679. Bibcode:1999Natur.399..676U. doi:10.1038/21406. ISSN 0028-0836.
- D Sinclair, W (2007-01-01). "Porphyry deposits". Geological Association of Canada, Mineral Deposits Division, Special Publication. 5: 223–243.
- Schwartz, George Melvin (1947-06-01). "Hydrothermal alteration in the "porphyry copper" deposits". Economic Geology. 42 (4): 319–352. doi:10.2113/gsecongeo.42.4.319. ISSN 1554-0774.
- Cooke, D. R. (2005-08-01). "Giant Porphyry Deposits: Characteristics, Distribution, and Tectonic Controls". Economic Geology. 100 (5): 801–818. doi:10.2113/gsecongeo.100.5.801. ISSN 0361-0128.
- Cooke, D. R. (2005-08-01). "Giant Porphyry Deposits: Characteristics, Distribution, and Tectonic Controls". Economic Geology. 100 (5): 801–818. doi:10.2113/gsecongeo.100.5.801. ISSN 0361-0128.
- Johnson, Douglas (2014-11-01). "The Nature and Origin of Pebble Dikes and Associated Alteration: Tintic Mining District (Ag-Pb-Zn), Utah". Theses and Dissertations.
- Taylor, R.D., Hammarstrom, J.M., Piatak, N.M., and Seal II, R.R., 2012, Arc-related porphyry molybdenum deposit model: Chapter D in Mineral deposit models for resource assessment: U.S. Geological Survey Scientific Investigations Report USGS Numbered Series 2010-5070-D, http://pubs.er.usgs.gov/publication/sir20105070D
- Edwards, Richard; Atkinson, Keith (1986), "Magmatic hydrothermal deposits", Ore Deposit Geology and its Influence on Mineral Exploration, Springer Netherlands, pp. 69–142, doi:10.1007/978-94-011-8056-6_3, ISBN 9789401180580
- Einaudi, Marco T.; Burt, Donald M. (1982-07-01). "Introduction; terminology, classification, and composition of skarn deposits". Economic Geology. 77 (4): 745–754. doi:10.2113/gsecongeo.77.4.745. ISSN 1554-0774.
- Williams-Jones, A. E. (2005-10-01). "100th Anniversary Special Paper: Vapor Transport of Metals and the Formation of Magmatic-Hydrothermal Ore Deposits". Economic Geology. 100 (7): 1287–1312. doi:10.2113/gsecongeo.100.7.1287. ISSN 0361-0128.
- Fitzpatrick, Harry M. (1919-04-18). "George Francis Atkinson". Science. 49 (1268): 371–372. Bibcode:1919Sci....49..371F. doi:10.1126/science.49.1268.371. ISSN 0036-8075. PMID 17730106.
- "The Meanings and Features of Skarns", W-Sn Skarn Deposits and Related Metamorphic Skarns and Granitoids, Developments in Economic Geology, 24, Elsevier, 1987, pp. 27–53, doi:10.1016/b978-0-444-42820-2.50008-0, ISBN 9780444428202
- Meinert, Lawrence D.; Hefton, Kristopher K.; Mayes, David; Tasiran, Ian (1997-08-01). "Geology, zonation, and fluid evolution of the Big Gossan Cu-Au skarn deposit, Ertsberg District, Irian Jaya". Economic Geology. 92 (5): 509–534. doi:10.2113/gsecongeo.92.5.509. ISSN 1554-0774.
- Rowland, J. V.; Simmons, S. F. (2012-04-04). "Hydrologic, Magmatic, and Tectonic Controls on Hydrothermal Flow, Taupo Volcanic Zone, New Zealand: Implications for the Formation of Epithermal Vein Deposits". Economic Geology. 107 (3): 427–457. doi:10.2113/econgeo.107.3.427. ISSN 0361-0128.
- Deb, Mihir; Sarkar, Sanjib Chandra (2017), "Energy Resources", Minerals and Allied Natural Resources and their Sustainable Development, Springer Singapore, pp. 351–419, doi:10.1007/978-981-10-4564-6_6, ISBN 9789811045639
- Lindgren, J. (1933). "Komet 1933 a (Peltier)". Astronomische Nachrichten. 249 (17): 307–308. doi:10.1002/asna.19332491705. ISSN 0004-6337.
- W., Hedenquist, J. (1996). Epithermal gold deposits : styles, characteristics, and exploration. Society of Resource Geology. OCLC 38057627.
- "Fluorspar Mines at St. Lawrence". www.virtualmuseum.ca.
- Schulz, K. J.; DeYoung, John H.; Seal, Robert R.; Bradley, Dwight C. (2018). Critical Mineral Resources of the United States: Economic and Environmental Geology and Prospects for Future Supply. Government Printing Office. p. S40. ISBN 9781411339910.
- Hannington, Mark D.; Jamieson, John; Petersen, Sven (May 2015). Seafloor massive sulfide deposits: Continuing efforts toward a global estimate of seafloor massive sulfides. OCEANS 2015 - Genova. IEEE. doi:10.1109/oceans-genova.2015.7271526. ISBN 9781479987368.
- Sangster, D F (1977). "Some Grade and Tonnage Relationships Among Canadian Volcanogenic Massive Sulphide Deposits". doi:10.4095/102647. Cite journal requires
|journal=
(help) - Davis, Franklin A.; et al. (2005-08-23). "Asymmetric Synthesis Using Sulfinimines (N-Sulfinyl Imines)". ChemInform. 36 (34). doi:10.1002/chin.200534036. ISSN 0931-7597.
- Herrington, Richard; Maslennikov, Valeriy; Zaykov, Victor; Seravkin, Igor; Kosarev, Alexander; Buschmann, Bernd; Orgeval, Jean-Jacques; Holland, Nicola; Tesalina, Svetlana (November 2005). "6: Classification of VMS deposits: Lessons from the South Uralides". Ore Geology Reviews. 27 (1–4): 203–237. doi:10.1016/j.oregeorev.2005.07.014. ISSN 0169-1368.
- Allen, Rodney L.; Weihed, Par; Svenson, Sven-Ake (1996-10-01). "Setting of Zn-Cu-Au-Ag massive sulfide deposits in the evolution and facies architecture of a 1.9 Ga marine volcanic arc, Skellefte District, Sweden". Economic Geology. 91 (6): 1022–1053. doi:10.2113/gsecongeo.91.6.1022. ISSN 1554-0774.
- Sangster, Donald F. (2001-10-17). "The role of dense brines in the formation of vent-distal sedimentary-exhalative (SEDEX) lead–zinc deposits: field and laboratory evidence". Mineralium Deposita. 37 (2): 149–157. doi:10.1007/s00126-001-0216-9. ISSN 0026-4598.
- Laznicka, Peter (2010), "From trace metals to giant deposits", Giant Metallic Deposits, Springer Berlin Heidelberg, pp. 59–68, doi:10.1007/978-3-642-12405-1_3, ISBN 9783642124044
- Taylor, Cliff D.; Johnson, Craig A. (2010). "Geology, geochemistry, and genesis of the Greens Creek massive sulfide deposit, Admiralty Island, southeastern Alaska". Professional Paper. doi:10.3133/pp1763. ISSN 2330-7102.
- Song, X. (1994), "Sediment-Hosted Pb-Zn Deposits in China: Mineralogy, Geochemistry and Comparison with Some Similar Deposits in the World", Sediment-Hosted Zn-Pb Ores, Springer Berlin Heidelberg, pp. 333–353, doi:10.1007/978-3-662-03054-7_18, ISBN 9783662030561
External links
![]() |
Look up hydrothermal mineral deposit in Wiktionary, the free dictionary. |