Native chemical ligation
Native chemical ligation or NCL is an important extension of the chemical ligation field, a concept for constructing a large polypeptide formed by the assembling of two or more unprotected peptides segments.[1] Especially, NCL is the most powerful ligation method for synthesizing native backbone proteins or modified proteins of moderate size (i.e., small proteins< 200 AA).
Reaction
In native chemical ligation, the thiol group of an N-terminal cysteine residue of an unprotected peptide 2 attacks the C-terminal thioester of a second unprotected peptide 1 in an aqueous buffer at pH 7.0, 20 °C<T<37 °C. This reversible transthioesterification step is chemoselective and regioselective and leads to form a thioester intermediate 3. This intermediate rearranges by an intramolecular S,N-acyl shift that results in the formation of a native amide ('peptide') bond 4 at the ligation site (scheme 1).
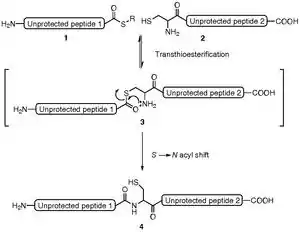
Remarks :
- Thiol additives :
NCL reaction is catalyzed by in situ transthioesterification with thiol additives. The most common thiol catalysts to date have been either a mixture of thiophenyl, 4-mercaptophenylacetic acid (MPAA), or 2-mercaptoethanesulfonate (MESNa). (ref)
- Regioselectivity of NCL :
The main property of the NCL method is the reversibility of the first step, the thiol(ate)–thioester exchange reaction. Native chemical ligation is exquisitely regioselective because that thiol(ate)–thioester exchange step is freely reversible in the presence of an exogenous thiol added as catalyst. The high yields of final ligation product obtained, even in the presence of internal Cys residues in either/both segments, is the result of the irreversibility, under the reaction conditions used, of the second (S-to-N acyl shift) amide-forming step.
- Chemoselectivity of NCL :
No side-products are formed from reaction with the other functional groups present in either peptide segment (acids or basics amino groups, phenolic hydroxyls, etc.).
Historical context
In 1953, Theodor Wieland and coworkers discovered the chemical foundation for this reaction, when the reaction of valine-thioester and cysteine amino acid in aqueous buffer was shown to yield the dipeptide valine-cysteine.[2] The reaction proceeded through the intermediacy of a thioester containing the sulfur of the cysteine residue. Wieland's work led to the 'active ester' method for making protected peptide segments in conventional solution synthesis in organic solvents.
In the 1990s, Stephen Kent and coworkers at The Scripps Research Institute independently developed "Native Chemical Ligation", the first practical method to ligate large unprotected peptide fragments.
Properties
Native chemical ligation is carried out in aqueous solution and frequently gives near-quantitative yields of the desired ligation product. The challenge lies in the preparation of the necessary unprotected peptide-thioester building block. Peptide-thioesters are usually prepared by Boc chemistry SPPS; a thioester-containing peptide cannot be synthesized using a nucleophilic base, thus disfavoring Fmoc chemistry. Fmoc chemistry solid phase peptide synthesis techniques for generating peptide-thioesters are known; they make use of modifications of the Kenner 'safety catch' linker. In making peptide segments for use in native chemical ligation, protecting groups that release aldehydes or ketones should be avoided since these may cap the N-terminal cysteine. For the same reason, the use of acetone should be avoided, particularly prior to lyophilization and in washing glassware.
A feature of the native chemical ligation technique is that the product polypeptide chain contains cysteine at the site of ligation. For some proteins, homocysteine can be used and methylated after ligation to form methionine, although side reactions can occur in this alkylation step. The cysteine at the ligation site can also be desulfurized to alanine; more recently, other beta-thiol containing aminoacids have been used for native chemical ligation, followed by desulfurization. Alternatively, thiol-containing ligation auxiliaries can be used that mimic an N-terminal cysteine for the ligation reaction, but which can be removed after synthesis. The use of thiol-containing auxiliaries is not as effective as ligation at a Cys residue. Native chemical ligation can also be performed with an N-terminal selenocysteine residue.[3]
The payoff in the native chemical ligation method is that coupling long peptides by this technique is in many cases nearly quantitative and provides synthetic access to large peptides and proteins otherwise impossible to make, due to length or decoration by post-translational modification. Native chemical ligation forms the basis of modern chemical protein synthesis, and has been used to prepare numerous proteins and enzymes by total chemical synthesis.
Polypeptide C-terminal thioesters produced by recombinant DNA techniques can be reacted with an N-terminal Cys containing polypeptide by the same native ligation chemistry to provide very large semi-synthetic proteins. Native chemical ligation of this kind using a recombinant polypeptide segment is known as Expressed Protein Ligation. Similarly, a recombinant protein containing an N-terminal Cys can be reacted with a synthetic polypeptide thioester. Thus, native chemical ligation can be used to introduce chemically synthesized segments into recombinant proteins, regardless of size.
Polypeptide C-terminal thioesters can also be produced in situ, using so-called N,S-acyl shift systems. Bis(2-sulfanylethyl)amido group, also called SEA group, belongs to this family. Polypeptide C-terminal bis(2-sulfanylethyl)amides (SEA peptide segments) react with Cys peptide to give a native peptide bond as in NCL. This reaction, which is called SEA Native Peptide Ligation, is a useful extension of Native Chemical Ligation.[4][5]
Size limitation
NCL has been proven to be a convenient method for the chemical synthesis of proteins well over 100 amino acids in length, as demonstrated on the 166-amino-acid polypeptide chain of the synthetic variant of erythropoietin and the 203 amino acid HIV-1 protease.
See also
- Intein
- KAHA Ligation
- Peptide synthesis
- Protein synthesis
- SEA Native Peptide Ligation
References
- Dawson, P. E.; Muir, T. W.; Clark-Lewis, I.; Kent, S. B. (1994). "Synthesis of proteins by native chemical ligation". Science. 266 (5186): 776–778. Bibcode:1994Sci...266..776D. doi:10.1126/science.7973629. PMID 7973629.
- Wieland, T.; Bokelmann, E.; Bauer, L.; Lang, H. U.; Lau, H (1953). "Über Peptidsynthesen. 8. Mitteilung Bildung von S-haltigen Peptiden durch intramolekulare Wanderung von Aminoacylresten". Liebigs Ann. Chem. 583: 129–149. doi:10.1002/jlac.19535830110.
- McGrath, N. A.; Raines, R. T. (2011). "Chemoselectivity in chemical biology: Acyl transfer reactions with sulfur and selenium". Acc. Chem. Res. 44 (9): 752–761. doi:10.1021/ar200081s. PMC 3242736. PMID 21639109.
- Ollivier, N. Dheur, J. Mhidia, R. Blanpain, A. Melnyk, O. (2010). "Bis(2-sulfanylethyl)amino Native Peptide Ligation". Organic Letters. 12 (22): 5238–41. doi:10.1021/ol102273u. PMID 20964289.CS1 maint: multiple names: authors list (link)
- Melnyk, O.; et al. "(WO2011051906) METHOD FOR NATIVE LIGATION OF POLYPEPTIDES". WO patent application.
Further reading
- Muir T. W.; Sondhi D.; Cole P. A. (1998). "Expressed protein ligation: A general method for protein engineering". Proc. Natl. Acad. Sci. USA. 95 (12): 6705–6710. Bibcode:1998PNAS...95.6705M. doi:10.1073/pnas.95.12.6705. PMC 22605. PMID 9618476.
- Nilsson B. L.; Soellner M. B.; Raines R. T. (2005). "Chemical synthesis of proteins". Annu. Rev. Biophys. Biomol. Struct. 34: 91–118. doi:10.1146/annurev.biophys.34.040204.144700. PMC 2845543. PMID 15869385.